Salvia miltiorrhiza extract dihydrotanshinone induces apoptosis and inhibits proliferation of glioma cells
DOI:
https://doi.org/10.17305/bjbms.2017.1800Keywords:
Dihydrotanshinone, glioma, SHG-44 cells, cell apoptosis, cell proliferation, MTT assayAbstract
Dihydrotanshinone, a functional food in China, is an effective anti-cardiovascular disease substance isolated from Salvia miltiorrhiza (S. miltiorrhiza). Glioma is considered to be fatal due to its poor prognosis and few effective therapeutic options. In this study, we investigated the anticancer effects of S. miltiorrhiza extract dihydrotanshinone on human glioma SHG-44 cells, by using 3-(4, 5-dimethyl-2-thiazolyl)-2, 5-diphenyl-2H-tetrazolium bromide assay, Hoechst 33258 nuclear staining, Annexin V/propidium iodide double staining, as well as western blot analysis. The results showed that dihydrotanshinone effectively suppressed SHG-44 cells proliferation and induced apoptosis in both dose- and time-dependent manner. Moreover, we demonstrated that dihydrotanshinone increased the activation of caspases (caspase-3 and caspase-9) and the release of cytochrome c in SHG-44 cells. Overall, dihydrotanshinone could induce apoptosis and inhibit proliferation of glioma cells by regulating caspases and cytochrome c. This study suggests that dihydrotanshinone may serve as a potential treatment option for patients with glioma.
Citations
Downloads
References
Tzen JT, Jinn TR, Chen YC, Li FY, Cheng FC, Shi LS, et al. Magnesium lithospermate B possesses inhibitory activity on Na+, K+ - ATPase and neuroprotective effects against ischemic stroke. Acta Pharmacol Sin 2007;28(5):609-15.
https://doi.org/10.1111/j.1745-7254.2007.00544.x.
Wang X, Morris-Natschke SL, Lee KH. New developments in the chemistry and biology of the bioactive constituents of Tanshen. Med Res Rev 2007;27(1):133-48. https://doi.org/10.1002/med.20077.
Tian XH, Wu JH. Tanshinone derivatives: A patent review (January 2006 - September 2012). Expert Opin Ther Pat 2013;23(1):19-29. https://doi.org/10.1517/13543776.2013.736494.
Franek KJ, Zhou Z, Zhang WD, Chen WY. In vitro studies of baicalin alone or in combination with Salvia miltiorrhiza extract as a potential anti-cancer agent. Int J Oncol 2005;26(1):217-24. https://doi.org/10.3892/ijo.26.1.217.
Chang JY, Chang CY, Kuo CC, Chen LT, Wein YS, Kuo YH. Salvinal, a novel microtubule inhibitor isolated from Salvia miltiorrhizae Bunge (Danshen), with antimitotic activity in multidrug-sensitive and -resistant human tumor cells. Mol Pharmacol 2004;65(1):77-84. https://doi.org/10.1124/mol.65.1.77.
Chen W, Lu Y, Chen G, Huang S. Molecular evidence of cryptotanshinone for treatment and prevention of human cancer. Anticancer Agents Med Chem 2013;13(7):979-87. https://doi.org/10.2174/18715206113139990115.
Bian W, Chen F, Bai L, Zhang P, Qin W. Dihydrotanshinone I inhibits angiogenesis both in vitro and in vivo. Acta Biochim Biophys Sin (Shanghai) 2008;40(1):1-6. https://doi.org/10.1111/j.1745-7270.2008.00370.x.
D'Agostino VG, Lal P, Mantelli B, Tiedje C, Zucal C, Thongon N, et al. Dihydrotanshinone-I interferes with the RNA-binding activity of HuR affecting its post-transcriptional function. Sci Rep 2015;5:16478. https://doi.org/10.1038/srep16478.
Lee DS, Lee SH. Biological activity of dihydrotanshinone I: Effect on apoptosis. J Biosci Bioeng 2000;89(3):292-3. https://doi.org/10.1016/S1389-1723(00)88838-6.
Chen MH, Jenh YJ, Wu SK, Chen YS, Hanagata N, Lin FH. Non-invasive photodynamic therapy in brain cancer by use of Tb3 - Doped LaF3 nanoparticles in combination with photosensitizer through X-ray irradiation: A proof-of-concept study. Nanoscale Res Lett 2017;12(1):62. https://doi.org/10.1186/s11671-017-1840-3.
Perry NS, Houghton PJ, Theobald A, Jenner P, Perry EK. In-vitro inhibition of human erythrocyte acetylcholinesterase by salvia lavandulaefolia essential oil and constituent terpenes. J Pharm Pharmacol 2000;52(7):895-902. https://doi.org/10.1211/0022357001774598.
Zhang JH, Xu M. DNA fragmentation in apoptosis. Cell Res 2000;10(3):205-11. https://doi.org/10.1038/sj.cr.7290049.
Kajstura M, Halicka HD, Pryjma J, Darzynkiewicz Z. Discontinuous fragmentation of nuclear DNA during apoptosis revealed by discrete "sub-G1" peaks on DNA content histograms. Cytometry A 2007;71(3):125-31. https://doi.org/10.1002/cyto.a.20357.
Barciszewska AM. MicroRNAs as efficient biomarkers in high-grade gliomas. Folia Neuropathol 2016;54(4):369-74. https://doi.org/10.5114/fn.2016.64812.
Hamza MA, Gilbert M. Targeted therapy in gliomas. Curr Oncol Rep 2014;16(4):379. https://doi.org/10.1007/s11912-014-0379-z.
Kaufmann JK, Chiocca EA. Glioma virus therapies between bench and bedside. Neuro Oncol 2014;16(3):334-51. https://doi.org/10.1093/neuonc/not310.
Zhuo Z, Hu J, Yang X, Chen M, Lei X, Deng L, et al. Ailanthone inhibits Huh7 cancer cell growth via cell cycle arrest and apoptosis in vitro and in vivo. Sci Rep 2015;5:16185. https://doi.org/10.1038/srep16185.
Liu JJ, Wu HH, Chen TH, Leung W, Liang YC. 15,16-dihydrotanshinone I from the functional food Salvia miltiorrhiza exhibits anticancer activity in human HL-60 leukemia cells: In vitro and in vivo studies. Int J Mol Sci 2015;16(8):19387-400. https://doi.org/10.3390/ijms160819387.
Saraste A, Pulkki K. Morphologic and biochemical hallmarks of apoptosis. Cardiovasc Res 2000;45(3):528-37. https://doi.org/10.1016/S0008-6363(99)00384-3.
Huang WW, Chiu YJ, Fan MJ, Lu HF, Yeh HF, Li KH, et al. Kaempferol induced apoptosis via endoplasmic reticulum stress and mitochondria-dependent pathway in human osteosarcoma U-2 OS cells. Mol Nutr Food Res 2010;54(11):1585-95. https://doi.org/10.1002/mnfr.201000005.
Alnemri ES, Livingston DJ, Nicholson DW, Salvesen G, Thornberry NA, Wong WW, et al. Human ICE/CED-3 protease nomenclature. Cell 1996;87(2):171. https://doi.org/10.1016/S0092-8674(00)81334-3.
Zou H, Henzel WJ, Liu X, Lutschg A, Wang X. Apaf-1, a human protein homologous to C. Elegans CED-4, participates in cytochrome c-dependent activation of caspase-3. Cell 1997;90(3):405-13. https://doi.org/10.1016/S0092-8674(00)80501-2.
Wolf BB, Green DR. Suicidal tendencies: Apoptotic cell death by caspase family proteinases. J Biol Chem 1999;274(29):20049-52. https://doi.org/10.1074/jbc.274.29.20049.
Tsai SL, Suk FM, Wang CI, Liu DZ, Hou WC, Lin PJ, et al. Anti-tumor potential of 15, 16-dihydrotanshinone I against breast adenocarcinoma through inducing G1 arrest and apoptosis. Biochem Pharmacol 2007;74(11):1575-86. https://doi.org/10.1016/j.bcp.2007.08.009.
Wang L, Hu T, Shen J, Zhang L, Chan RL, Lu L, et al. Dihydrotanshinone I induced apoptosis and autophagy through caspase dependent pathway in colon cancer. Phytomedicine 2015;22(12):1079-87. https://doi.org/10.1016/j.phymed.2015.08.009.
Lee WY, Liu KW, Yeung JH. Reactive oxygen species-mediated kinase activation by dihydrotanshinone in tanshinones-induced apoptosis in HepG2 cells. Cancer Lett 2009;285(1):46-57. https://doi.org/10.1016/j.canlet.2009.04.040.
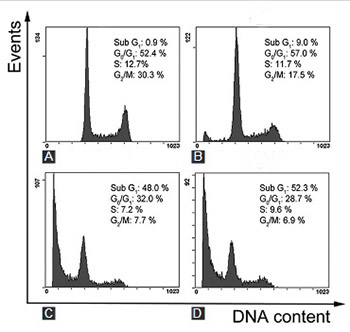