The effect of 131I-induced hypothyroidism on the levels of nitric oxide (NO), interleukin 6 (IL-6), tumor necrosis factor alpha (TNF-α), total nitric oxide synthase (NOS) activity, and expression of NOS isoforms in rats
DOI:
https://doi.org/10.17305/bjbms.2018.2350Keywords:
Hypothyroidism, I-131, radioactive iodine, aorta, nitric oxide, NO, nitric oxide synthase, NOS, eNOS, nNOS, iNOS, inflammatory mediators, interleukin, IL-6, TNF-αAbstract
Accumulating evidence has shown that hypothyroidism affects the cardiovascular system, significantly increasing the incidence of cardiovascular diseases. In the present study we investigated the effect of radioactive iodine (I-131)-induced hypothyroidism on several parameters of vascular function, such as nitric oxide (NO), total nitric oxide synthase (NOS) activity and expression of NOS isoforms, as well as on interleukin 6 (IL-6) and tumor necrosis factor alpha (TNF-α) as indicators of inflammation, in rats. A dose of 150 µCi of 131-I was determined as optimal for establishing the model of hypothyroidism in rats. After administration of 131-I, at the end of month 1, 2, and 4 (n = 3 for each time point), NO, IL-6, and TNF-α in the serum and total NOS activity in the aorta were determined in 150 µCi group, compared to controls. The mRNA and protein expression of endothelial, neuronal, and inducible NOS (eNOS, nNOS, and iNOS) in the rat aorta was also estimated, using quantitative reverse transcription polymerase chain reaction and Western blot, respectively. The levels of IL-6 and TNF-α increased in 150 µCi group; the results were significant at the end of month 2 and 4 for IL-6, and at all time points for TNF-α. The levels of NO decreased significantly at the end of month 2 and 4 in 150 µCi group. The total NOS activity increased significantly in 150 µCi group, at all three time points. Significant changes in the mRNA and protein expression of all three NOS isoforms were observed in 150 µCi group compared to controls. NO, IL-6, TNF-α levels and NOS activity and expression are altered in hypothyroid state, and the underlying mechanism should be further investigated.
Citations
Downloads
References
Lu ZY, Zhong NS. Internal Medicine. 7th ed. Beijing: People's Medical Publishing House; 2008.
Aizawa Y, Yoshida K, Kaise N, Fukazawa H, Kiso Y, Sayama N, et al. The development of transient hypothyroidism after iodine-131 treatment in hyperthyroid patients with Graves' disease: Prevalence, mechanism and prognosis. Clin Endocrinol (Oxf) 1997;46(1):1-5. https://doi.org/10.1046/j.1365-2265.1997.d01-1737.x.
Alexander EK, Larsen PR. High dose of (131)I therapy for the treatment of hyperthyroidism caused by Graves' disease. J Clin Endocrinol Metab 2002;87(3):1073-7. https://doi.org/10.1210/jcem.87.3.8333.
Gomez N, Gomez JM, Orti A, Gavalda L, Villabona C, Leyes P, et al. Transient hypothyroidism after iodine-131 therapy for Grave's disease. J Nucl Med 1995;36(9):1539-42.
Canaris GJ, Manowitz NR, Mayor G, Ridgway EC. The Colorado thyroid disease prevalence study. Arch Intern Med 2000;160(4):526-34. https://doi.org/10.1001/archinte.160.4.526.
Biondi B, Palmieri EA, Lombardi G, Fazio S. Effects of subclinical thyroid dysfunction on the heart. Ann Intern Med 2002;137(11):904-14.
https://doi.org/10.7326/0003-4819-137-11-200212030-00011.
Cappola AR, Ladenson PW. Hypothyroidism and atherosclerosis. J Clin Endocrinol Metab 2003;88(6):2438-44. https://doi.org/10.1210/jc.2003-030398.
Klein I, Ojamaa K. Thyroid hormone and the cardiovascular system. N Engl J Med 2001;344(7):501-9. https://doi.org/10.1056/NEJM200102153440707.
Vargas F, Moreno JM, Rodriguez-Gomez I, Wangensteen R, Osuna A, Alvarez-Guerra M, et al. Vascular and renal function in experimental thyroid disorders. Eur J Endocrinol 2006;154(2):197-212. https://doi.org/10.1530/eje.1.02093.
Flammer AJ, Luscher TF. Three decades of endothelium research: From the detection of nitric oxide to the everyday implementation of endothelial function measurements in cardiovascular diseases. Swiss Med Wkly 2010;140:w13122. https://doi.org/10.4414/smw.2010.13122.
Luscher TF, Vanhoutte PM. The Endothelium: Modulator of Cardiovascular function. Florida, USA: CRC press; 1990.
Förstermann U, Sessa WC. Nitric oxide synthases: regulation and function. Eur Heart J 2012;33(7):829-37,837a-837d. https://doi.org/10.1093/eurheartj/ehr304.
Tsutsui M, Shimokawa H, Otsuji Y, Ueta Y, Sasaguri Y, Yanagihara N. Nitric oxide synthases and cardiovascular diseases: Insights from genetically modified mice. Circ J 2009; 73(6):986-93. https://doi.org/10.1253/circj.CJ-09-0208.
Abbas AM, Sakr HF. Effect of magnesium sulfate and thyroxine on inflammatory markers in a rat model of hypothyroidism. Can J Physiol Pharmacol 2016;94(4):426-32. https://doi.org/10.1139/cjpp-2015-0247.
Hajje G, Saliba Y, Itani T, Moubarak M, Aftimos G, Fares N. Hypothyroidism and its rapid correction alter cardiac remodeling. PLoS One 2014;9(10):e109753. https://doi.org/10.1371/journal.pone.0109753.
Diez JJ, Hernanz A, Medina S, Bayon C, Iglesias P. Serum concentrations of tumour necrosis factor-alpha (TNF-alpha) and soluble TNF-alpha receptor p55 in patients with hypothyroidism and hyperthyroidism before and after normalization of thyroid function. Clin Endocrinol (Oxf) 2002;57(4):515-21. https://doi.org/10.1046/j.1365-2265.2002.01629.x.
Johnson C, Olivier NB, Nachreiner R, Mullaney T. Effect of 131I-induced hypothyroidism on indices of reproductive function in adult male dogs. J Vet Intern Med 1999;13(2):104-10. https://doi.org/10.1892/0891-6640(1999)013<0104:EOIHOI>2.3.CO;2.
Pasteur I, Tronko N, Drozdovich I, Donich S, Voitenko L. Xenotransplantation of cultured newborn pig thyroid tissue for the treatment of post-radioiodine hypothyroidism in rats. Cytotechnology 2000;33(1-3):89-92. https://doi.org/10.1023/A:1008185701106.
Reilly CP, Symons RG, Wellby ML. A rat model of the 131I-induced changes in thyroid function. J Endocrinol Invest 1986;9(5):367-70. https://doi.org/10.1007/BF03346944.
Torlak V, Zemunik T, Modun D, Capkun V, Pesutic-Pisac V, Markotic A, et al. 131 I-induced changes in rat thyroid gland function. Braz J Med Biol Res 2007;40(8):1087-94. https://doi.org/10.1590/S0100-879X2006005000127.
Hosseini M, Dastghaib SS, Rafatpanah H, Hadjzadeh MA, Nahrevanian H, Farrokhi I. Nitric oxide contributes to learning and memory deficits observed in hypothyroid rats during neonatal and juvenile growth. Clinics (Sao Paulo) 2010;65(11):1175-81. https://doi.org/10.1590/S1807-59322010001100021.
McAllister RM, Albarracin I, Price EM, Smith TK, Turk JR, Wyatt KD. Thyroid status and nitric oxide in rat arterial vessels. J Endocrinol 2005;185(1):111-9. https://doi.org/10.1677/joe.1.06022.
Quesada A, Sainz J, Wangensteen R, Rodriguez-Gomez I, Vargas F, Osuna A. Nitric oxide synthase activity in hyperthyroid and hypothyroid rats. Eur J Endocrinol 2002;147(1): 117-22. https://doi.org/10.1530/eje.0.1470117.
Engin AB, Sepici-Dincel A, Gonul II, Engin A. Oxidative stress-induced endothelial cell damage in thyroidectomized rat. Exp Toxicol Pathol 2012;64(5):481-5. https://doi.org/10.1016/j.etp.2010.11.002.
Dizdarevic-Bostandic A, Burekovic A, Velija-Asimi Z, Godinjak A. Inflammatory markers in patients with hypothyroidism and diabetes mellitus type 1. Med Arch 2013;67(3):160-1. https://doi.org/10.5455/medarh.2013.67.160-161.
Marfella R, Ferraraccio F, Rizzo MR, Portoghese M, Barbieri M, Basilio C, et al. Innate immune activity in plaque of patients with untreated and L-thyroxine-treated subclinical hypothyroidism. J Clin Endocrinol Metab 2011;96(4):1015-20. https://doi.org/10.1210/jc.2010-1382.
Guida MS, Abd El-Aal A, Kafafy Y, Salama SF, Badr BM, Badr G. Thymoquinone rescues T lymphocytes from gamma irradiation-induced apoptosis and exhaustion by modulating pro-inflammatory cytokine levels and PD-1, Bax, and Bcl-2 signaling. Cell Physiol Biochem 2016;38(2):786-800. https://doi.org/10.1159/000443034.
Koukkunen H, Penttila K, Kemppainen A, Halinen M, Penttila I, Rantanen T, et al. C-reactive protein, fibrinogen, interleukin-6 and tumour necrosis factor-alpha in the prognostic classification of unstable angina pectoris. Ann Med 2001;33(1):37-47. https://doi.org/10.3109/07853890109002058.
Gao C, Li T, Liu J, Guo Q, Tian L. Endothelial functioning and hemodynamic parameters in rats with subclinical hypothyroid and the effects of thyroxine replacement. PLoS One 2015;10:e0131776. https://doi.org/10.1371/journal.pone.0131776.
Ibuki Y, Goto R. Ionizing radiation-induced macrophage activation: Augmentation of nitric oxide production and its significance. Cell Mol Biol (Noisy-le-grand) 2004;50 Online Pub:OL617-626.
Lacoste-Collin L, Jozan S, Pereda V, Courtade-Saidi M. Influence of a continuous very low dose of gamma-rays on cell proliferation, apoptosis and oxidative stress. Dose Response 2015;13(1): dose-response.14-010.Lacoste-Collin.
https://doi.org/10.2203/dose-response.14-010.Lacoste-Collin.
Nowosielska EM, Wrembel-Wargocka J, Cheda A, Lisiak E, Janiak MK. Enhanced cytotoxic activity of macrophages and suppressed tumor metastases in mice irradiated with low doses of X- rays. J Radiat Res 2006;47(3-4):229-36. https://doi.org/10.1269/jrr.0572.
Sarati LI, Martinez CR, Artes N, Arreche N, Lopez-Costa JJ, Balaszczuk AM, et al. Hypothyroidism: Age-related influence on cardiovascular nitric oxide system in rats. Metabolism 2012;61(9):1301-11. https://doi.org/10.1016/j.metabol.2012.01.022.
Fellet AL, Arza P, Arreche N, Arranz C, Balaszczuk AM. Nitric oxide and thyroid gland: Modulation of cardiovascular function in autonomic-blocked anaesthetized rats. Exp Physiol 2004;89(3):303-12. https://doi.org/10.1113/expphysiol.2004.027201.
Rodriguez-Gomez I, Moliz JN, Quesada A, Montoro-Molina S, Vargas-Tendero P, Osuna A, et al. L-Arginine metabolism in cardiovascular and renal tissue from hyper- and hypothyroid rats. Exp Biol Med (Maywood) 2016;241(5):550-6. https://doi.org/10.1177/1535370215619042.
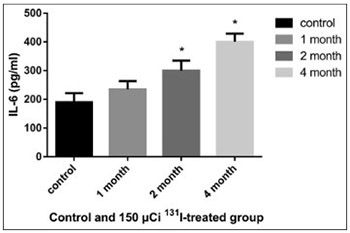
Downloads
Additional Files
Published
How to Cite
Accepted 2017-10-26
Published 2018-11-07