Cholesteryl ester transfer protein, low density lipoprotein particle size and intima media thickness in patients with coronary heart disease
DOI:
https://doi.org/10.17305/bjbms.2011.2569Keywords:
cholesteryl ester transfer protein, coronary heart disease, intima media thickness, reverse cholesterol transportAbstract
Cholesteryl ester transfer protein (CETP) plays a key role in reverse cholesterol transport and high density lipoprotein (HDL) metabolism. Predominance of small, dense LDL particles is associated with an increased risk of atherosclerosis and coronary heart disease (CHD).
The aim of the study was to determine the potential relationship between the CETP concentration and low density lipoprotein (LDL) particle size and their association with intima media thickness (IMT) in patients with CHD. Lipid parameters, CETP concentration and LDL particle size were determined in 100 healthy subjects (control group) and in 100 patients with CHD, aged 43 to 77 years. Plasma CETP concentrations were measured by an enzyme-linked immuno-sorbent assay with two different monoclonal antibodies. LDL subclasses were separated by nondenaturing polyacrilamide 3-31% gradient gel electrophoresis. CETP concentration was higher in patients compared to controls (2.02 ± 0.75 mg/ml vs. 1.74 ± 0.63 mg/ml, p<0.01). Mean LDL particle size (nm) was significantly smaller in patients than in controls (24.5 ± 1.1 vs. 26.1 ± 0.9; p<0.001). There was no relation between LDL particle size and CETP concentration (r=-0.1807, p=0.072). Age, diastolic blood pressure, CETP concentration and LDL particle size were independent factors for determing IMT by multiple linear regression analysis. They accounted for 35.2 % of the observed variability in IMT. CETP is not an independent contributor of LDL particle size. CETP might play a role in determining lipoprotein distributions, but did not seem to be the sole factor in the formation of small LDL particles.
Citations
Downloads
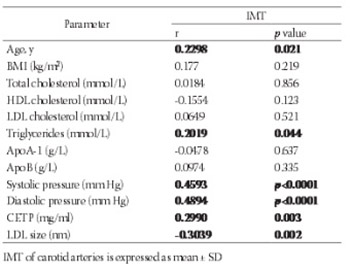
Downloads
Additional Files
Published
Issue
Section
Categories
How to Cite
Accepted 2017-10-16
Published 2011-08-20