The role of the Hedgehog signaling pathway in cancer: A comprehensive review
DOI:
https://doi.org/10.17305/bjbms.2018.2756Keywords:
Hedgehog signaling pathway, Hh, tumorigenesis, signal transductionAbstract
The Hedgehog (Hh) signaling pathway was first identified in the common fruit fly. It is a highly conserved evolutionary pathway of signal transmission from the cell membrane to the nucleus. The Hh signaling pathway plays an important role in the embryonic development. It exerts its biological effects through a signaling cascade that culminates in a change of balance between activator and repressor forms of glioma-associated oncogene (Gli) transcription factors. The components of the Hh signaling pathway involved in the signaling transfer to the Gli transcription factors include Hedgehog ligands (Sonic Hh [SHh], Indian Hh [IHh], and Desert Hh [DHh]), Patched receptor (Ptch1, Ptch2), Smoothened receptor (Smo), Suppressor of fused homolog (Sufu), kinesin protein Kif7, protein kinase A (PKA), and cyclic adenosine monophosphate (cAMP). The activator form of Gli travels to the nucleus and stimulates the transcription of the target genes by binding to their promoters. The main target genes of the Hh signaling pathway are PTCH1, PTCH2, and GLI1. Deregulation of the Hh signaling pathway is associated with developmental anomalies and cancer, including Gorlin syndrome, and sporadic cancers, such as basal cell carcinoma, medulloblastoma, pancreatic, breast, colon, ovarian, and small-cell lung carcinomas. The aberrant activation of the Hh signaling pathway is caused by mutations in the related genes (ligand-independent signaling) or by the excessive expression of the Hh signaling molecules (ligand-dependent signaling – autocrine or paracrine). Several Hh signaling pathway inhibitors, such as vismodegib and sonidegib, have been developed for cancer treatment. These drugs are regarded as promising cancer therapies, especially for patients with refractory/advanced cancers.
Citations
Downloads
References
Varjosalo M, Taipale J. Hedgehog: Functions and mechanisms. Genes Dev 2008;22(18):2454-72. https://doi.org/10.1101/gad.1693608.
Le H, Kleinerman R, Lerman OZ, Brown D, Galiano R, Gurtner GC, et al. Hedgehog signaling is essential for normal wound healing. Wound Repair Regen 2008;16(6):768-73. https://doi.org/10.1111/j.1524-475X.2008.00430.x.
Lowry WE, Richter L, Yachechko R, Pyle AD, Tchieu J, Sridharan R, et al. Generation of human induced pluripotent stem cells from dermal fibroblasts. Proc Natl Acad Sci U S A 2008;105(8):2883-8. https://doi.org/10.1073/pnas.0711983105.
Lewis MT, Veltmaat JM. Next top, the twilight zone: Hedgehog network regulation of mammary gland development. J Mammary Gland Biol Neoplasia 2004;9(2):165-81. https://doi.org/10.1023/B:JOMG.0000037160.24731.35.
Zhou JX, Jia LW, Liu WM, Miao CL, Liu S, Cao YJ, et al. Role of sonic hedgehog in maintaining a pool of proliferating stem cells in the human fetal epidermis. Hum Reprod 2006;21(7):1698-704. https://doi.org/10.1093/humrep/del086.
Stecca B, Mas C, Clement V, Zbinden M, Correa R, Piguet V, et al. Melanomas require HEDGEHOG-GLI signaling regulated by interactions between GLI1 and the RAS-MEK/AKT pathways. Proc Natl Acad Sci U S A 2007;104(14):5895-900. https://doi.org/10.1073/pnas.0700776104.
Detmer K, Thompson AJ, Garner RE, Walker AN, Gaffield W, Dannawi H, et al. Hedgehog signaling and cell cycle control in differentiating erythroid progenitors. Blood Cells Mol Dis 2005;34(1):60-70. https://doi.org/10.1016/j.bcmd.2004.08.026.
Watkins DN, Berman DM, Burkholder SG, Wang B, Beachy PA, Baylin SB. Hedgehog signalling within airway epithelial progenitors and in small-cell lung cancer. Nature 2003;422(6929):313-7. https://doi.org/10.1038/nature01493.
Beachy PA, Karhadkar SS, Berman DM. Tissue repair and stem cell renewal in carcinogenesis. Nature 2004;432(7015):324-31. https://doi.org/10.1038/nature03100.
Karhadkar SS, Bova GS, Abdallah N, Dhara S, Gardner D, Maitra A, et al. Hedgehog signalling in prostate regeneration, neoplasia and metastasis. Nature 2004;431(7009):707-12. https://doi.org/10.1038/nature02962.
Fendrich V, Esni F, Garay MV, Feldmann G, Habbe N, Jensen JN, et al. Hedgehog signaling is required for effective regeneration of exocrine pancreas. Gastroenterology 2008;135(2):621-31. https://doi.org/10.1053/j.gastro.2008.04.011.
Plotnikova OV, Golemis EA, Pugacheva EN. Cell cycle-dependent ciliogenesis and cancer. Cancer Res 2008;68(7):2058-61. https://doi.org/10.1158/0008-5472.CAN-07-5838.
Michaud EJ, Yoder BK. The primary cilium in cell signaling and cancer. Cancer Res 2006;66(13):6463-7. https://doi.org/10.1158/0008-5472.CAN-06-0462.
Bailey JM, Mohr AM, Hollingsworth MA. Sonic hedgehog paracrine signaling regulates metastasis and lymphangiogenesis in pancreatic cancer. Oncogene 2009;28(40):3513-25. https://doi.org/10.1038/onc.2009.220.
Ma X, Sheng T, Zhang Y, Zhang X, He J, Huang S, et al. Hedgehog signaling is activated in subsets of esophageal cancers. Int J Cancer 2006;118(1):139-48. https://doi.org/10.1002/ijc.21295.
Sheng T, Li C, Zhang X, Chi S, He N, Chen K, et al. Activation of the hedgehog pathway in advanced prostate cancer. Mol Cancer 2004;3:29.
https://doi.org/10.1186/1476-4598-3-29.
Cross SS, Bury JP. The Hedgehog signalling pathways in human pathology. Curr Diagnostic Pathol 2004;10(2):157-68. https://doi.org/10.1016/j.cdip.2003.11.005.
Denef N, Neubüser D, Perez L, Cohen SM. Hedgehog induces opposite changes in turnover and subcellular localization of patched and smoothened. Cell 2000;102(4):521-31. https://doi.org/10.1016/S0092-8674(00)00056-8.
Price MA, Kalderon D. Proteolysis of the hedgehog signaling effector cubitus interruptus requires phosphorylation by glycogen synthase kinase 3 and casein kinase 1. Cell 2002;108(6):823-35. https://doi.org/10.1016/S0092-8674(02)00664-5.
Goetz SC, Anderson KV. The primary cilium: A signalling centre during vertebrate development. Nat Rev Genet 2010;11(5):331-44. https://doi.org/10.1038/nrg2774.
Mastronardi FG, Dimitroulakos J, Kamel-Reid S, Manoukian AS. Co-localization of patched and activated sonic hedgehog to lysosomes in neurons. Neuroreport 2000;11(3):581-5. https://doi.org/10.1097/00001756-200002280-00030.
Rubin LL, de Sauvage FJ. Targeting the hedgehog pathway in cancer. Nat Rev Drug Discov 2006;5(12):1026-33. https://doi.org/10.1038/nrd2086.
Echelard Y, Epstein DJ, St-Jacques B, Shen L, Mohler J, McMahon JA, et al. Sonic hedgehog, a member of a family of putative signaling molecules, is implicated in the regulation of CNS polarity. Cell 1993;75(7):1417-30.
https://doi.org/10.1016/0092-8674(93)90627-3.
Krauss S, Concordet JP, Ingham PW. A functionally conserved homolog of the drosophila segment polarity gene hh is expressed in tissues with polarizing activity in zebrafish embryos. Cell 1993;75(7):1431-44. https://doi.org/10.1016/0092-8674(93)90628-4.
Marigo V, Tabin CJ. Regulation of patched by sonic hedgehog in the developing neural tube. Proc Natl Acad Sci U S A 1996;93(18):9346-51. https://doi.org/10.1073/pnas.93.18.9346.
Pathi S, Pagan-Westphal S, Baker DP, Garber EA, Rayhorn P, Bumcrot D, et al. Comparative biological responses to human sonic, indian, and desert hedgehog. Mech Dev 2001;106(1-2):107-17. https://doi.org/10.1016/S0925-4773(01)00427-0.
Goodrich LV, Johnson RL, Milenkovic L, McMahon JA, Scott MP. Conservation of the hedgehog/patched signaling pathway from flies to mice: Induction of a mouse patched gene by hedgehog. Genes Dev 1996;10(3):301-12. https://doi.org/10.1101/gad.10.3.301.
Bellusci S, Furuta Y, Rush MG, Henderson R, Winnier G, Hogan BL, et al. Involvement of sonic hedgehog (Shh) in mouse embryonic lung growth and morphogenesis. Development 1997;124(1):53-63.
Hardcastle Z, Mo R, Hui CC, Sharpe PT. The shh signalling pathway in tooth development: Defects in gli2 and gli3 mutants. Development 1998;125(15):2803-11.
Litingtung Y, Lei L, Westphal H, Chiang C. Sonic hedgehog is essential to foregut development. Nat Genet 1998;20(1):58-61. https://doi.org/10.1038/1717.
Vortkamp A, Lee K, Lanske B, Segre GV, Kronenberg HM, Tabin CJ, et al. Regulation of rate of cartilage differentiation by indian hedgehog and PTH-related protein. Science 1996;273(5275):613-22. https://doi.org/10.1126/science.273.5275.613.
van den Brink GR, Bleuming SA, Hardwick JC, Schepman BL, Offerhaus GJ, Keller JJ, et al. Indian hedgehog is an antagonist of wnt signaling in colonic epithelial cell differentiation. Nat Genet 2004;36(3):277-82. https://doi.org/10.1038/ng1304.
Robbins DJ, Fei DL, Riobo NA. The hedgehog signal transduction network. Sci Signal 2012;5(246):re6. https://doi.org/10.1126/scisignal.2002906.
Bitgood MJ, Shen L, McMahon AP. Sertoli cell signaling by desert hedgehog regulates the male germline. Curr Biol 1996;6(3):298-304.
https://doi.org/10.1016/S0960-9822(02)00480-3.
Johnson RL, Scott MP. New players and puzzles in the hedgehog signaling pathway. Curr Opin Genet Dev 1998;8(4):450-6. https://doi.org/10.1016/S0959-437X(98)80117-2.
Mann RK, Beachy PA. Novel lipid modifications of secreted protein signals. Annu Rev Biochem 2004;73:891-923. https://doi.org/10.1146/annurev.biochem.73.011303.073933.
Buglino JA, Resh MD. Hhat is a palmitoylacyltransferase with specificity for N-palmitoylation of sonic hedgehog. J Biol Chem 2008;283(32):22076-88. https://doi.org/10.1074/jbc.M803901200.
Zaphiropoulos PG, Undén AB, Rahnama F, Hollingsworth RE, Toftgård R. PTCH2, a novel human patched gene, undergoing alternative splicing and up-regulated in basal cell carcinomas. Cancer Res 1999;59(3):787-92.
Gailani MR, Ståhle-Bäckdahl M, Leffell DJ, Glynn M, Zaphiropoulos PG, Pressman C, et al. The role of the human homologue of Drosophila patched in sporadic basal cell carcinomas. Nat Genet 1996;14(1):78-81. https://doi.org/10.1038/ng0996-78.
Johnson RL, Rothman AL, Xie J, Goodrich LV, Bare JW, Bonifas JM, et al. Human homolog of patched, a candidate gene for the basal cell nevus syndrome. Science 1996;272(5268):1668-71. https://doi.org/10.1126/science.272.5268.1668.
Hahn H, Christiansen J, Wicking C, Zaphiropoulos PG, Chidambaram A, Gerrard B, et al. A mammalian patched homolog is expressed in target tissues of sonic hedgehog and maps to a region associated with developmental abnormalities. J Biol Chem 1996;271(21):12125-8. https://doi.org/10.1074/jbc.271.21.12125.
Smyth I, Narang MA, Evans T, Heimann C, Nakamura Y, Chenevix-Trench G, et al. Isolation and characterization of human patched 2 (PTCH2), a putative tumour suppressor gene inbasal cell carcinoma and medulloblastoma on chromosome 1p32. Hum Mol Genet 1999;8(2):291-7. https://doi.org/10.1093/hmg/8.2.291.
Larsson NG, Wang J, Wilhelmsson H, Oldfors A, Rustin P, Lewandoski M, et al. Mitochondrial transcription factor A is necessary for mtDNA maintenance and embryogenesis in mice. Nat Genet 1998;18(3):231-6. https://doi.org/10.1038/ng0398-231.
Ingham PW. How cholesterol modulates the signal. Curr Biol 2000;10(5):R180-3. https://doi.org/10.1016/S0960-9822(00)00346-8.
Ingham PW, Taylor AM, Nakano Y. Role of the Drosophila patched gene in positional signalling. Nature 1991;353(6340):184-7. https://doi.org/10.1038/353184a0.
Marigo V, Davey RA, Zuo Y, Cunningham JM, Tabin CJ. Biochemical evidence that patched is the hedgehog receptor. Nature 1996;384(6605):176-9. https://doi.org/10.1038/384176a0.
Johnson RL, Milenkovic L, Scott MP. In vivo functions of the patched protein: Requirement of the C terminus for target gene inactivation but not hedgehog sequestration. Mol Cell 2000;6(2):467-78. https://doi.org/10.1016/S1097-2765(00)00045-9.
Taylor AM, Nakano Y, Mohler J, Ingham PW. Contrasting distributions of patched and hedgehog proteins in the drosophila embryo. Mech Dev 1993;42(1-2):89-96. https://doi.org/10.1016/0925-4773(93)90101-3.
Chen Y, Struhl G. Dual roles for patched in sequestering and transducing hedgehog. Cell 1996;87(3):553-63. https://doi.org/10.1016/S0092-8674(00)81374-4.
Fan H, Khavari PA. Sonic hedgehog opposes epithelial cell cycle arrest. J Cell Biol 1999;147(1):71-6. https://doi.org/10.1083/jcb.147.1.71.
Duman-Scheel M, Weng L, Xin S, Du W. Hedgehog regulates cell growth and proliferation by inducing cyclin D and cyclin E. Nature 2002;417:299-304. https://doi.org/10.1038/417299a.
Bhat KM, Schedl P. Requirement for engrailed and invected genes reveals novel regulatory interactions between engrailed/invected, patched, gooseberry and wingless during Drosophila neurogenesis. Development 1997;124(9):1675-88.
Ramírez-Weber FA, Casso DJ, Aza-Blanc P, Tabata T, Kornberg TB. Hedgehog signal transduction in the posterior compartment of the Drosophila wing imaginal disc. Mol Cell 2000;6(2):479-85. https://doi.org/10.1016/S1097-2765(00)00046-0.
Chuang PT, McMahon AP. Vertebrate hedgehog signalling modulated by induction of a hedgehog-binding protein. Nature 1999;397(6720):617-21. https://doi.org/10.1038/17611.
Tenzen T, Allen BL, Cole F, Kang JS, Krauss RS, McMahon AP, et al. The cell surface membrane proteins cdo and boc are components and targets of the hedgehog signaling pathway and feedback network in mice. Dev Cell 2006;10(5):647-56. https://doi.org/10.1016/j.devcel.2006.04.004.
Martinelli DC, Fan CM. Gas1 extends the range of hedgehog action by facilitating its signaling. Genes Dev 2007;21(10):1231-43. https://doi.org/10.1101/gad.1546307.
Jeong J, McMahon AP. Growth and pattern of the mammalian neural tube are governed by partially overlapping feedback activities of the hedgehog antagonists patched 1 and hhip1. Development 2005;132(1):143-54. https://doi.org/10.1242/dev.01566.
Alcedo J, Ayzenzon M, Von Ohlen T, Noll M, Hooper JE. The Drosophila smoothened gene encodes a seven-pass membrane protein, a putative receptor for the hedgehog signal. Cell 1996;86(2):221-32. https://doi.org/10.1016/S0092-8674(00)80094-X.
Rana R, Carroll CE, Lee HJ, Bao J, Marada S, Grace CR, et al. Structural insights into the role of the smoothened cysteine-rich domain in hedgehog signalling. Nat Commun 2013;4:2965. https://doi.org/10.1038/ncomms3965.
Murone M, Rosenthal A, de Sauvage FJ. Hedgehog signal transduction: From flies to vertebrates. Exp Cell Res 1999;253(1):25-33. https://doi.org/10.1006/excr.1999.4676.
Stone DM, Hynes M, Armanini M, Swanson TA, Gu Q, Johnson RL, et al. The tumour-suppressor gene patched encodes a candidate receptor for sonic hedgehog. Nature 1996;384(6605):129-34. https://doi.org/10.1038/384129a0.
Taipale J, Cooper MK, Maiti T, Beachy PA. Patched acts catalytically to suppress the activity of smoothened. Nature 2002;418(6900):892-7. https://doi.org/10.1038/nature00989.
Kim J, Hsia EY, Brigui A, Plessis A, Beachy PA, Zheng X, et al. The role of ciliary trafficking in hedgehog receptor signaling. Sci Signal 2015;8(379):ra55. https://doi.org/10.1126/scisignal.aaa5622.
Corcoran RB, Scott MP. Oxysterols stimulate sonic hedgehog signal transduction and proliferation of medulloblastoma cells. Proc Natl Acad Sci U S A 2006;103(22):8408-13. https://doi.org/10.1073/pnas.0602852103.
Bijlsma MF, Spek CA, Zivkovic D, van de Water S, Rezaee F, Peppelenbosch MP, et al. Repression of smoothened by patched-dependent (pro-)vitamin D3 secretion. PLoS Biol 2006;4(8):e232. https://doi.org/10.1371/journal.pbio.0040232.
Jia J, Tong C, Wang B, Luo L, Jiang J. Hedgehog signalling activity of smoothened requires phosphorylation by protein kinase A and casein kinase I. Nature 2004;432(7020):1045-50. https://doi.org/10.1038/nature03179.
Kinzler KW, Bigner SH, Bigner DD, Trent JM, Law ML, O'Brien SJ, et al. Identification of an amplified, highly expressed gene in a human glioma. Science 1987;236(4797):70-3. https://doi.org/10.1126/science.3563490.
Hui CC, Slusarski D, Platt KA, Holmgren R, Joyner AL. Expression of three mouse homologs of the Drosophila segment polarity gene cubitus interruptus, gli, gli-2, and gli-3, in ectoderm-and mesoderm-derived tissues suggests multiple roles during postimplantation development. Dev Biol 1994;162(2):402-13. https://doi.org/10.1006/dbio.1994.1097.
Hynes M, Stone DM, Dowd M, Pitts-Meek S, Goddard A, Gurney A, et al. Control of cell pattern in the neural tube by the zinc finger transcription factor and oncogene gli-1. Neuron 1997;19(1):15-26. https://doi.org/10.1016/S0896-6273(00)80344-X.
Regl G, Neill GW, Eichberger T, Kasper M, Ikram MS, Koller J, et al. Human GLI2 and GLI1 are part of a positive feedback mechanism in basal cell carcinoma. Oncogene 2002;21(36):5529-39. https://doi.org/10.1038/sj.onc.1205748.
Yoon JW, Liu CZ, Yang JT, Swart R, Iannaccone P, Walterhouse D, et al. GLI activates transcription through a herpes simplex viral protein 16-like activation domain. J Biol Chem 1998;273(6):3496-501. https://doi.org/10.1074/jbc.273.6.3496.
Persson M, Stamataki D, te Welscher P, Andersson E, Böse J, Rüther U, et al. Dorsal-ventral patterning of the spinal cord requires gli3 transcriptional repressor activity. Genes Dev 2002;16(22):2865-78. https://doi.org/10.1101/gad.243402.
Sasaki H, Hui C, Nakafuku M, Kondoh H. A binding site for gli proteins is essential for HNF-3beta floor plate enhancer activity in transgenics and can respond to shh in vitro. Development 1997;124(7):1313-22.
Kinzler KW, Vogelstein B. The GLI gene encodes a nuclear protein which binds specific sequences in the human genome. Mol Cell Biol 1990;10(2):634-42. https://doi.org/10.1128/MCB.10.2.634.
Tanimura A, Dan S, Yoshida M. Cloning of novel isoforms of the human gli2 oncogene and their activities to enhance tax-dependent transcription of the human T-cell leukemia virus Type 1 genome. J Virol 1998;72(5):3958-64.
Pan Y, Bai CB, Joyner AL, Wang B. Sonic hedgehog signaling regulates gli2 transcriptional activity by suppressing its processing and degradation. Mol Cell Biol 2006;26(9):3365-77. https://doi.org/10.1128/MCB.26.9.3365-3377.2006.
Humke EW, Dorn KV, Milenkovic L, Scott MP, Rohatgi R. The output of hedgehog signaling is controlled by the dynamic association between suppressor of fused and the gli proteins. Genes Dev 2010;24(7):670-82. https://doi.org/10.1101/gad.1902910.
Wang Y, Ding Q, Yen CJ, Xia W, Izzo JG, Lang JY, et al. The crosstalk of mTOR/S6K1 and hedgehog pathways. Cancer Cell 2012;21(3):374-87. https://doi.org/10.1016/j.ccr.2011.12.028.
Mimeault M, Rachagani S, Muniyan S, Seshacharyulu P, Johansson SL, Datta K, et al. Inhibition of hedgehog signaling improves the anti-carcinogenic effects of docetaxel in prostate cancer. Oncotarget 2015;6(6):3887-903. https://doi.org/10.18632/oncotarget.2932.
Stone DM, Murone M, Luoh S, Ye W, Armanini MP, Gurney A, et al. Characterization of the human suppressor of fused, a negative regulator of the zinc-finger transcription factor gli. J Cell Sci 1999;112:4437-48.
Tukachinsky H, Lopez LV, Salic A. A mechanism for vertebrate hedgehog signaling: Recruitment to cilia and dissociation of SuFu-Gli protein complexes. J Cell Biol 2010;191(2):415-28. https://doi.org/10.1083/jcb.201004108.
Méthot N, Basler K. Suppressor of fused opposes hedgehog signal transduction by impeding nuclear accumulation of the activator form of cubitus interruptus. Development 2000;127(18):4001-10.
Chen M, Wilson CW, Li Y, Ruel L, Thérond PP, King K, et al. Cilium-independent regulation of gli protein function by sufu in hedgehog signaling is evolutionarily conserved. Genes Dev 2009;23(16):1910-28. https://doi.org/10.1101/gad.1794109.
Liem KF Jr., He M, Ocbina PJ, Anderson KV. Mouse kif7/Costal2 is a cilia-associated protein that regulates sonic hedgehog signaling. Proc Natl Acad Sci U S A 2009;106(32):13377-82. https://doi.org/10.1073/pnas.0906944106.
He M, Subramanian R, Bangs F, Omelchenko T, Liem KF Jr., Kapoor TM, et al. The kinesin-4 protein kif7 regulates mammalian hedgehog signalling by organizing the cilium tip compartment. Nat Cell Biol 2014;16(7):663-72. https://doi.org/10.1038/ncb2988.
Haycraft CJ, Banizs B, Aydin-Son Y, Zhang Q, Michaud EJ, Yoder BK, et al. Gli2 and gli3 localize to cilia and require the intraflagellar transport protein polaris for processing and function. PLoS Genet 2005;1(4):e53. https://doi.org/10.1371/journal.pgen.0010053.
Liu YC, Couzens AL, Deshwar AR, McBroom-Cerajewski LD, Zhang X, Puviindran V, et al. The PPFIA1-PP2A protein complex promotes trafficking of kif7 to the ciliary tip and hedgehog signaling. Sci Signal 2014;7(355):ra117. https://doi.org/10.1126/scisignal.2005608.
Jiang J, Struhl G. Protein kinase A and hedgehog signaling in Drosophila limb development. Cell 1995;80(4):563-72. https://doi.org/10.1016/0092-8674(95)90510-3.
Barzi M, Berenguer J, Menendez A, Alvarez-Rodriguez R, Pons S. Sonic-hedgehog-mediated proliferation requires the localization of PKA to the cilium base. J Cell Sci 2010;123(1):62-9. https://doi.org/10.1242/jcs.060020.
Wang B, Fallon JF, Beachy PA. Hedgehog-regulated processing of gli3 produces an anterior/posterior repressor gradient in the developing vertebrate limb. Cell 2000;100(4):423-34. https://doi.org/10.1016/S0092-8674(00)80678-9.
Li W, Ohlmeyer JT, Lane ME, Kalderon D. Function of protein kinase a in hedgehog signal transduction and Drosophila imaginal disc development. Cell 1995;80(4):553-62. https://doi.org/10.1016/0092-8674(95)90509-X.
Dai P, Akimaru H, Tanaka Y, Maekawa T, Nakafuku M, Ishii S, et al. Sonic hedgehog-induced activation of the gli1 promoter is mediated by GLI3. J Biol Chem 1999;274(12):8143-52. https://doi.org/10.1074/jbc.274.12.8143.
Bonifas JM, Pennypacker S, Chuang PT, McMahon AP, Williams M, Rosenthal A, et al. Activation of expression of hedgehog target genes in basal cell carcinomas. J Invest Dermatol 2001;116(5):739-42. https://doi.org/10.1046/j.1523-1747.2001.01315.x.
Bigelow RL, Chari NS, Unde AB, Spurgers KB, Lee S, Roop DR, et al. Transcriptional regulation of bcl-2 mediated by the sonic hedgehog signaling pathway through gli-1. J Biol Chem 2004;279:1197-205. https://doi.org/10.1074/jbc.M310589200.
Oliver TG, Grasfeder LL, Carroll AL, Kaiser C, Gillingham CL, Lin SM, et al. Transcriptional profiling of the sonic hedgehog response: A critical role for N-myc in proliferation of neuronal precursors. Proc Natl Acad Sci U S A 2003;100(12):7331-6. https://doi.org/10.1073/pnas.0832317100.
Singh RR, Kunkalla K, Qu C, Schlette E, Neelapu SS, Samaniego F, et al. ABCG2 is a direct transcriptional target of hedgehog signaling and involved in stroma-induced drug tolerance in diffuse large B-cell lymphoma. Oncogene 2011;30(49):4874-86. https://doi.org/10.1038/onc.2011.195.
Bouldin CM, Harfe BD. Aberrant FGF signaling, independent of ectopic hedgehog signaling, initiates preaxial polydactyly in dorking chickens. Dev Biol 2009;334(1):133-41. https://doi.org/10.1016/j.ydbio.2009.07.009.
Morrow D, Cullen JP, Liu W, Guha S, Sweeney C, Birney YA, et al. Sonic hedgehog induces notch target gene expression in vascular smooth muscle cells via VEGF-A. Arterioscler Thromb Vasc Biol 2009;29(7):1112-8. https://doi.org/10.1161/ATVBAHA.109.186890.
Macdonald R, Barth K, Xu Q, Holder N, Mikkola I, Wilson SW. Midline signalling is required for pax gene regulation and pattering of the eyes. Development 1995;121(10):3267-78.
Teh MT, Wong ST, Neill GW, Ghali LR, Philpott MP, Quinn AG, et al. FOXM1 is a downstream target of gli1 in basal cell carcinomas. Cancer Res 2002;62(16):4773-80.
Katoh M, Katoh M. Notch ligand, JAG1, is evolutionarily conserved target of canonical WNT signaling pathway in progenitor cells. Int J Mol Med 2006;17(4):681-5. https://doi.org/10.3892/ijmm.17.4.681.
Mullor JL, Dahmane N, Sun T, Ruiz i Altaba A. Wnt signals are targets and mediators of gli function. Curr Biol 2001;11(10):769-73.
https://doi.org/10.1016/S0960-9822(01)00229-9.
Taipale J, Beachy PA. The hedgehog and wnt signalling pathways in cancer. Nature 2001;411(6835):349-54. https://doi.org/10.1038/35077219.
Hahn H, Wicking C, Zaphiropoulous PG, Gailani MR, Shanley S, Chidambaram A, et al. Mutations of the human homolog of Drosophila patched in the nevoid basal cell carcinoma syndrome. Cell 1996;85(6):841-51. https://doi.org/10.1016/S0092-8674(00)81268-4.
Reifenberger J, Wolter M, Knobbe CB, Köhler B, Schönicke A, Scharwächter C, et al. Somatic mutations in the PTCH, SMOH, SUFUH and TP53 genes in sporadic basal cell carcinomas. Br J Dermatol 2005;152(1):43-51.
https://doi.org/10.1111/j.1365-2133.2005.06353.x.
Johnson RL, Rothman AL, Xie J, Goodrich LV, Bare JW, Bonifas JM, et al. Human homolog of patched, a candidate gene for the basal cell nevus syndrome. Science 1996;272(5268):1668-71. https://doi.org/10.1126/science.272.5268.1668.
Dahmane N, Lee J, Robins P, Heller P, Ruiz i Altaba A. Activation of the transcription factor gli1 and the sonic hedgehog signalling pathway in skin tumours. Nature 1997;389(6653):876-81. https://doi.org/10.1038/39918.
Aszterbaum M, Epstein J, Oro A, Douglas V, LeBoit PE, Scott MP, et al. Ultraviolet and ionizing radiation enhance the growth of BCCs and trichoblastomas in patched heterozygous knockout mice. Nat Med 1999;5(11):1285-91. https://doi.org/10.1038/15242.
Lee Y, Kawagoe R, Sasai K, Li Y, Russell HR, Curran T, et al. Loss of suppressor-of-fused function promotes tumorigenesis. Oncogene 2007;26(44):6442-7. https://doi.org/10.1038/sj.onc.1210467.
Kool M, Jones DT, Jäger N, Northcott PA, Pugh TJ, Hovestadt V, et al. Genome sequencing of SHH medulloblastoma predicts genotype-related response to smoothened inhibition. Cancer Cell 2014;25(3):393-405. https://doi.org/10.1016/j.ccr.2014.02.004.
Lo Muzio L. Nevoid basal cell carcinoma syndrome (Gorlin syndrome). Orphanet J Rare Dis 2008;3(32):1-16. DOI: 10.1186/1750-1172-3-32.
Romer JT, Kimura H, Magdaleno S, Sasai K, Fuller C, Baines H, et al. Suppression of the shh pathway using a small molecule inhibitor eliminates medulloblastoma in ptc1(+/-)p53(-/-) mice. Cancer Cell 2004;6(3):229-40. https://doi.org/10.1016/j.ccr.2004.08.019.
Raffel C, Jenkins RB, Frederick L, Hebrink D, Alderete B, Fults DW, et al. Sporadic medulloblastomas contain PTCH mutations. Cancer Res 1997;57(5):842-5.
Taylor MD, Liu L, Raffel C, Hui CC, Mainprize TG, Zhang X, et al. Mutations in SUFU predispose to medulloblastoma. Nat Genet 2002;31(3):306-10. https://doi.org/10.1038/ng916.
Iglesias-Bartolome R, Torres D, Marone R, Feng X, Martin D, Simaan M, et al. Inactivation of a Gαs–PKA tumour suppressor pathway in skin stem cells initiates basal-cell carcinogenesis. Nat Cell Biol 2015;17(6):793-803. https://doi.org/10.1038/ncb3164.
Vorechovský I, Undén AB, Sandstedt B, Toftgård R, Ståhle-Bäckdahl M. Trichoepitheliomas contain somatic mutations in the overexpressed PTCH gene: Support for a gatekeeper mechanism in skin tumorigenesis. Cancer Res 1997;57(21):4677-81.
Maesawa C, Tamura G, Iwaya T, Ogasawara S, Ishida K, Sato N, et al. Mutations in the human homologue of the Drosophila patched gene in esophageal squamous cell carcinoma. Genes Chromosomes Cancer 1998;21(3):276-9.
https://doi.org/10.1002/(SICI)1098-2264(199803)21:3<276::AID-GCC15>3.0.CO;2-N.
McGarvey TW, Maruta Y, Tomaszewski JE, Linnenbach AJ, Malkowicz SB. PTCH gene mutations in invasive transitional cell carcinoma of the bladder. Oncogene 1998;17(9):1167-72. https://doi.org/10.1038/sj.onc.1202045.
Almazán-Moga A, Zarzosa P, Molist C, Velasco P, Pyczek J, Simon-Keller K, et al. Ligand-dependent Hedgehog pathway activation in rhabdomyosarcoma: The oncogenic role of the ligands. Br J Cancer 2017;117(9):1314-25. https://doi.org/10.1038/bjc.2017.305.
Berman DM, Karhadkar SS, Maitra A, Montes De Oca R, Gerstenblith MR, Briggs K, et al. Widespread requirement for hedgehog ligand stimulation in growth of digestive tract tumours. Nature 2003;425(6960):846-51. https://doi.org/10.1038/nature01972.
Gulino A, Ferretti E, De Smaele E. Hedgehog signalling in colon cancer and stem cells. EMBO Mol Med 2009;1(6-7):300-2. https://doi.org/10.1002/emmm.200900042.
Szkandera J, Kiesslich T, Haybaeck J, Gerger A, Pichler M. Hedgehog signaling pathway in ovarian cancer. Int J Mol Sci 2013;14(1):1179-96. https://doi.org/10.3390/ijms14011179.
Kubo M, Nakamura M, Tasaki A, Yamanaka N, Nakashima H, Nomura M, et al. Hedgehog signaling pathway is a new therapeutic target for patients with breast cancer. Cancer Res 2004;64(17):6071-4. https://doi.org/10.1158/0008-5472.CAN-04-0416.
O'Reilly KE, de Miera EV, Segura MF, Friedman E, Poliseno L, Han SW, et al. Hedgehog pathway blockade inhibits melanoma cell growth in vitro and in vivo. Pharmaceuticals (Basel) 2013;6(11):1429-50. https://doi.org/10.3390/ph6111429.
Becher OJ, Hambardzumyan D, Fomchenko EI, Momota H, Mainwaring L, Bleau AM, et al. Gli activity correlates with tumor grade in platelet-derived growth factor-induced gliomas. Cancer Res 2008;68(7):2241-9. https://doi.org/10.1158/0008-5472.CAN-07-6350.
Monzo M, Moreno I, Artells R, Ibeas R, Navarro A, Moreno J, et al. Sonic hedgehog mRNA expression by real-time quantitative PCR in normal and tumor tissues from colorectal cancer patients. Cancer Lett 2006;233(1):117-23. https://doi.org/10.1016/j.canlet.2005.03.001.
Douard R, Moutereau S, Pernet P, Chimingqi M, Allory Y, Manivet P, et al. Sonic hedgehog-dependent proliferation in a series of patients with colorectal cancer. Surgery 2006;139(5):665-70. https://doi.org/10.1016/j.surg.2005.10.012.
van den Brink GR, Bleuming SA, Hardwick JC, Schepman BL, Offerhaus GJ, Keller JJ, et al. Indian hedgehog is an antagonist of wnt signaling in colonic epithelial cell differentiation. Nat Genet 2004;36(3):277-82. https://doi.org/10.1038/ng1304.
Akiyoshi T, Nakamura M, Koga K, Nakashima H, Yao T, Tsuneyoshi M, et al. Gli1, downregulated in colorectal cancers, inhibits proliferation of colon cancer cells involving wnt signalling activation. Gut 2006;55(7):991-9. https://doi.org/10.1136/gut.2005.080333.
Ingham PW, McMahon AP. Hedgehog signaling in animal development: Paradigms and principles. Genes Dev 2001;15(23):3059-87. https://doi.org/10.1101/gad.938601.
Jiang J, Hui CC. Hedgehog signaling in development and cancer. Dev Cell 2008;15(6):801-12. https://doi.org/10.1016/j.devcel.2008.11.010.
Fan L, Pepicelli CV, Dibble CC, Catbagan W, Zarycki JL, Laciak R, et al. Hedgehog signaling promotes prostate xenograft tumor growth. Endocrinology 2004;145(8):3961-70. https://doi.org/10.1210/en.2004-0079.
Theunissen J, Sauvage FJ De. Paracrine hedgehog signaling in cancer. Cancer Res 2009;69(15):6007-11. https://doi.org/10.1158/0008-5472.CAN-09-0756.
Read TA, Fogarty MP, Markant SL, McLendon RE, Wei Z, Ellison DW, et al. Identification of CD15 as a marker for tumor-propagating cells in a mouse model of medulloblastoma. Cancer Cell 2009;15(2):135-47. https://doi.org/10.1016/j.ccr.2008.12.016.
Zhao C, Chen A, Jamieson CH, Fereshteh M, Abrahamsson A, Blum J, et al. Hedgehog signalling is essential for maintenance of cancer stem cells in myeloid leukaemia. Nature 2009;458(7239):776-9. https://doi.org/10.1038/nature07737.
Nicolis SK. Cancer stem cells and “stemness” genes in neuro-oncology. Neurobiol Dis 2007;25(2):217-29. https://doi.org/10.1016/j.nbd.2006.08.022.
Peacock CD, Wang Q, Gesell GS, Corcoran-Schwartz IM, Jones E, Kim J, et al. Hedgehog signaling maintains a tumor stem cell compartment in multiple myeloma. Proc Natl Acad Sci U S A 2007;104(10):4048-53. https://doi.org/10.1073/pnas.0611682104.
Dembinski JL, Krauss S. Characterization and functional analysis of a slow cycling stem cell-like subpopulation in pancreas adenocarcinoma. Clin Exp Metastasis 2009;26(7):611-23. https://doi.org/10.1007/s10585-009-9260-0.
Liu S, Dontu G, Mantle ID, Patel S, Ahn NS, Jackson KW, et al. Hedgehog signaling and bmi-1 regulate self-renewal of normal and malignant human mammary stem cells. Cancer Res 2006;66(12):6063-71. https://doi.org/10.1158/0008-5472.CAN-06-0054.
Long B, Zhu H, Zhu C, Liu T, Meng W. Activation of the hedgehog pathway in chronic myelogeneous leukemia patients. J Exp Clin Cancer Res 2011;30(1):8. https://doi.org/10.1186/1756-9966-30-8.
Lou H, Dean M. Targeted therapy for cancer stem cells: The patched pathway and ABC transporters. Oncogene 2007;26(9):1357-60. https://doi.org/10.1038/sj.onc.1210200.
Cretnik M, Musani V, Oreskovic S, Leovic D, Levanat S. The patched gene is epigenetically regulated in ovarian dermoids and fibromas, but not in basocellular carcinomas. Int J Mol Med 2007;19(6):875-83. https://doi.org/10.3892/ijmm.19.6.875.
Wolf I, Bose S, Desmond JC, Lin BT, Williamson EA, Karlan BY, et al. Unmasking of epigenetically silenced genes reveals DNA promoter methylation and reduced expression of PTCH in breast cancer. Breast Cancer Res Treat 2007;105(2):139-55. https://doi.org/10.1007/s10549-006-9440-4.
Shi X, Zhang Z, Zhan X, Cao M, Satoh T, Akira S, et al. An epigenetic switch induced by Shh signalling regulates gene activation during development and medulloblastoma growth. Nat Commun 2014;5:5425. https://doi.org/10.1038/ncomms6425.
Huang S, He J, Zhang X, Bian Y, Yang L, Xie G, et al. Activation of the hedgehog pathway in human hepatocellular carcinomas. Carcinogenesis 2006;27(7):1334-40. https://doi.org/10.1093/carcin/bgi378.
Lauressergues E, Heusler P, Lestienne F, Troulier D, Rauly-Lestienne I, Tourette A, et al. Pharmacological evaluation of a series of smoothened antagonists in signaling pathways and after topical application in a depilated mouse model. Pharmacol Res Perspect 2016;4(2):e00214. DOI: 10.1002/prp2.214.
Yang L, Xie G, Fan Q, Xie J. Activation of the hedgehog-signaling pathway in human cancer and the clinical implications. Oncogene 2010;29(4):469-81. https://doi.org/10.1038/onc.2009.392.
Low JA, de Sauvage FJ. Clinical experience with hedgehog pathway inhibitors. J Clin Oncol 2010;28(36):5321-6. https://doi.org/10.1200/JCO.2010.27.9943.
Goel V, Hurh E, Stein A, Nedelman J, Zhou J, Chiparus O, et al. Population pharmacokinetics of sonidegib (LDE225), an oral inhibitor of hedgehog pathway signaling, in healthy subjects and in patients with advanced solid tumors. Cancer Chemother Pharmacol 2016;77(4):745-55. https://doi.org/10.1007/s00280-016-2982-1.
Basset-Seguin N, Sharpe HJ, de Sauvage FJ. Efficacy of hedgehog pathway inhibitors in basal cell carcinoma. Mol Cancer Ther 2015;14(3):633-41.
https://doi.org/10.1158/1535-7163.MCT-14-0703.
Kim J, Aftab BT, Tang JY, Kim D, Lee AH, Rezaee M, et al. Itraconazole and arsenic trioxide inhibit hedgehog pathway activation and tumor growth associated with acquired resistance to smoothened antagonists. Cancer Cell 2013;23(1):23-34. https://doi.org/10.1016/j.ccr.2012.11.017.
Long J, Li B, Rodriguez-Blanco J, Pastori C, Volmar CH, Wahlestedt C, et al. The BET bromodomain inhibitor I-BET151 acts downstream of smoothened protein to abrogate the growth of hedgehog protein-driven cancers. J Biol Chem 2014;289(51):35494-502. https://doi.org/10.1074/jbc.M114.595348.
Ge X, Milenkovic L, Suyama K, Hartl T, Purzner T, Winans A, et al. Phosphodiesterase 4D acts downstream of neuropilin to control hedgehog signal transduction and the growth of medulloblastoma. Elife 2015;4:e07068. https://doi.org/10.7554/eLife.07068.
Atwood SX, Li M, Lee A, Tang JY, Oro AE. GLI activation by atypical protein kinase C ι/λ regulates the growth of basal cell carcinomas. Nature 2013;494(7438):484-8. https://doi.org/10.1038/nature11889.
Mehta R, Katta H, Alimirah F, Patel R, Murillo G, Peng X, et al. Deguelin action involves c-met and EGFR signaling pathways in triple negative breast cancer cells. PLoS One 2013;8(6):e65113. https://doi.org/10.1371/journal.pone.0065113.
Cho YR, Choi SW, Seo DW. The in vitro antitumor activity of Siegesbeckia glabrescens against ovarian cancer through suppression of receptor tyrosine kinase expression and the signaling pathways. Oncol Rep 2013;30(1):221-6. https://doi.org/10.3892/or.2013.2468.
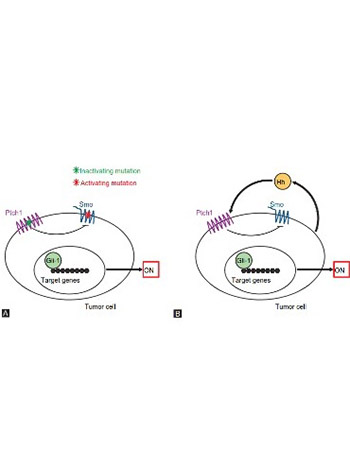