Prediction of breast cancer metastasis risk using circulating tumor markers: A follow-up study
DOI:
https://doi.org/10.17305/bjbms.2018.3371Keywords:
Breast cancer, circulating tumor cell markers, metastasisAbstract
Distant organ tumor dissemination is a major cause of breast cancer-related deaths. In 2010, we analyzed the prognostic importance of the circulating tumor markers (CTMs) cytokeratin 19 (CK19), CK20, epidermal growth factor receptor (EGFR) and human epidermal growth factor receptor 2 (HER2) in relation to the clinical and pathological characteristics of patients with breast cancer (BC). To assess the clinical utility of CK19, CK20 and EGFR in predicting distant metastasis in BC, here we report 7-year follow-up results of 77 patients. The patients with at least one positive CTM were classified as CTM(+) and those negative for all CTMs were assigned to CTM(-) group. In patients who received no treatment following CTM analysis, 25.0% had metastasis in CTM(+) and 10.0% in CTM(-) group. In patients who received one of the following therapies: chemotherapy, radiotherapy or hormone therapy, or the combinations of these therapies, the rate of metastasis was 33.3% in CTM(+) and 20.0% in CTM(-) group. Disease-free time was shorter in CTM(+) patients compared to CTM(-) group (28.83 ± 10.76 and 41.38 ± 9.5 months, respectively). According to multivariate Cox proportional hazard regression analysis, the presence of regional lymph node metastasis, Ki-67 expression, higher tumor grade and CTM expression status were predictors of poor prognosis associated with distant metastasis (p < 0.05). Also, CTM positivity was a factor associated with metastasis-related poor prognosis (HR = 0.492, p = 0.026). The mean survival for CTM(+) patients was shorter than that for CTM(-) patients (90.671 ± 2.66 and 101.23 ± 3.92 months, respectively; p > 0.05). Our findings demonstrate that CTM positivity may indicate a high metastasis risk; however, CTM negativity does not guarantee low metastasis risk. These results may encourage further preclinical investigation of CTMs, to evaluate the possible implications of these findings to the clinical setting.
Citations
Downloads
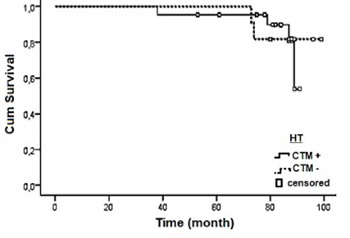
Downloads
Additional Files
Published
Issue
Section
Categories
How to Cite
Accepted 2018-06-28
Published 2019-05-20