Neurostructural correlates of strength decrease following total knee arthroplasty: A systematic review of the literature with meta-analysis
DOI:
https://doi.org/10.17305/bjbms.2019.3814Keywords:
knee replacement, functional performance, rehabilitation, activation failure, total knee arthroplasty, quadriceps muscle weakness, TKA, VMA, CSA, MVSAbstract
Recent literature suggests that alterations in both neural and structural components of the neuromuscular system are major determinants of knee extensor muscle weakness after total knee arthroplasty (TKA). Therefore, the goal of this study was to investigate the maximal voluntary strength (MVS), voluntary muscle activation (VMA), and the cross-sectional area (CSA) of the muscle, up to 33 months after the TKA. We searched relevant scientific databases and literature for outcomes of interest, including quadriceps MVS, VMA, and CSA. Ten studies met the inclusion criteria and involved a total of 289 patients. The quality of the studies was evaluated by Methodological Index for Non-Randomized Studies (MINORS). Results showed that quadriceps MVS markedly declines in the early postoperative period, after which it slowly and linearly recovers over time. However, the same phenomenon was not observed for VMA and CSA, which were not significantly altered after the TKA. Furthermore, a meta-regression analysis revealed that the change in VMA accounted for 39% of the relative change in quadriceps strength (R2=0.39; p=0.015) in the early postoperative period. Patients treated with TKA had considerable weakness of the quadriceps muscle, which was detectable up to 3 months after surgery. Although the change in VMA largely explains quadriceps weakness, this change and CSA differences were not significant, suggesting that other neural correlates, such as hamstrings coactivation, might alter quadriceps muscle function. Thus, more attention should be paid to address VMA failure and coactivation of antagonist muscles. More comprehensive rehabilitation approaches may be required to target the whole neural circuit controlling the motor action.
Citations
Downloads
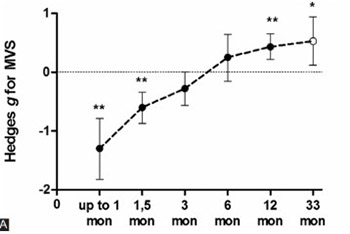
Downloads
Additional Files
Published
How to Cite
Accepted 2018-09-25
Published 2020-02-05