Recombinant deoxyribonucleoside kinase from Drosophila melanogaster can improve gemcitabine based combined gene/chemotherapy for targeting cancer cells
DOI:
https://doi.org/10.17305/bjbms.2019.4136Keywords:
Drosophila melanogaster deoxyribonucleoside kinase, suicide gene therapy, breast cancer, drug resistance, gemcitabineAbstract
A recombinant deoxyribonucleoside kinase from Drosophila melanogaster with a deletion of the last 20 amino acid residues (named DmdNKΔC20) was hypothesized as a potential therapeutic tool for gene therapy due to its broad substrate specificity and better catalytic efficiency towards nucleosides and nucleoside analogs. This study was designed to evaluate the effect of DmdNKΔC20 for sensitizing human cancer cell lines to gemcitabine and to further investigate its role in reversal of acquired drug resistance in gemcitabine-resistant cancer cell line. The DmdNKΔC20 gene was delivered to three different cancer cell lines, including breast, colon and liver cancer cells, using lipid-mediated transfection reagent. After transfection, gene expression of DmdNKΔC20 was confirmed by quantitative reverse transcription PCR (qRT-PCR) and the combined effect of DmdNKΔC20 and gemcitabine based cytotoxicity was observed by cell viability assay. We further evolved a gemcitabine-resistant breast cancer cell line (named MCF7-R) through directed evolution in the laboratory, which showed 375-fold more resistance compared with parental MCF7 cells. Upon transfection with DmdNKΔC20 gene, MCF7-R cells showed 83-fold higher sensitivity to gemcitabine compared with the control group of MCF7-R cells. Moreover, we observed 79% higher expression of p21 protein in transfected MCF7-R cells, which may indicate induction of apoptosis. Our findings highlight the importance and therapeutic potential of DmdNKΔC20 in combined gene/chemotherapy approach to target a wide range of cancers, particularly gemcitabine-resistant cancers.
Citations
Downloads
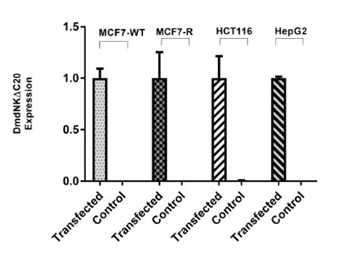
Downloads
Additional Files
Published
Issue
Section
Categories
How to Cite
Accepted 2019-03-03
Published 2019-11-08