Knockdown of TPT1-AS1 inhibits cell proliferation, cell cycle G1/S transition, and epithelial–mesenchymal transition in gastric cancer
DOI:
https://doi.org/10.17305/bjbms.2020.4470Keywords:
Gastric cancer, TPT1-AS1, prognosis, G1/S transition, epithelial– mesenchymal transition, EMTAbstract
Long non-coding RNAs are considered to be critical regulators of tumor progression. Tumor protein translationally controlled 1 antisense RNA 1 (TPT1-AS1) was shown to have an oncogenic role in cervical and ovarian cancer. The clinical significance and biological function of TPT1-AS1 in gastric cancer (GC) are not clear. In this study, we analyzed the expression of TPT1-AS1 in GC tissues and cell lines and performed functional and mechanistic analysis of TPT1-AS1 effects on GC cell proliferation, migration, and invasion. TPT1-AS1 expression was determined in 76 pairs of GC tissues vs. matched adjacent normal tissues and in four GC cell lines (SGC-7901, AGS, BGC-823, and MGC-803) vs. GES-1 cell line by quantitative reverse transcription PCR. SGC-7901 and MGC-803 cells were transfected with small interfering RNA or scrambled negative control, and cell proliferation, colony formation, migration, invasion and cell cycle assays were performed. The expression of proteins involved in cell cycle progression and epithelial–mesenchymal transition was analyzed by Western blot. TPT1-AS1 expression was significantly higher in GC tissues and cell lines compared to controls. The overexpression of TPT1-AS1 was significantly correlated with TNM stage and lymph node metastasis, and it was associated with worse prognosis of GC patients according to the Kaplan–Meier survival analysis and Cox proportional hazard regression analysis. The knockdown of TPT1-AS1 significantly inhibited proliferation, cell cycle G1/S transition, migration, and invasion of SGC-7901 and MGC-803 cells. Moreover, TPT1-AS1 knockdown downregulated the expression of cyclin-dependent kinase (CDK) 4, cyclin D1, and vimentin and upregulated the expression of p21 and E-cadherin. Our findings suggest that TPT1-AS1 may be a promising therapeutic target in GC.
Citations
Downloads
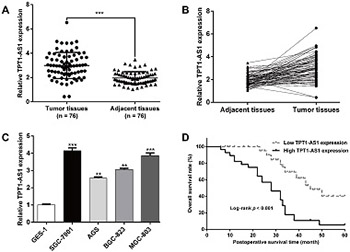