LncRNA SENCR suppresses abdominal aortic aneurysm formation by inhibiting smooth muscle cells apoptosis and extracellular matrix degradation
DOI:
https://doi.org/10.17305/bjbms.2020.4994Keywords:
Abdominal aortic aneurysm, lncRNA SENCR, vascular smooth muscle cells, cell apoptosis, extracellular matrix degradationAbstract
Abdominal aortic aneurysm (AAA) is a progressive chronic dilatation of the abdominal aorta without effective medical treatment. This study aims to clarify the potential of long non-coding RNA SENCR as a treatment target in AAA. Angiotensin II (Ang-II) was used to establish AAA model in vitro and in vivo. Reverse transcription quantitative PCR and western blot were performed to measure the expression of SENCR and proteins, respectively. Annexin V-FITC/PI double staining was carried out to detect the apoptotic rate in vascular smooth muscle cells (VSMCs), and cell apoptosis in aortic tissues was determined by TUNEL staining. Besides, hematoxylin and eosin and Elastica van Gieson staining were performed for histological analysis of aortic tissues. SENCR was downregulated in AAA tissues and Ang-II-stimulated VSMCs. Overexpression of SENCR could inhibit Ang-II-induced VSMC apoptosis, while inhibition of SENCR facilitated Ang-II-induced VSMC apoptosis. Moreover, the expression of matrix metalloproteinase (MMP)-2 and MMP-9 in Ang-II-induced VSMCs was reduced following SENCR overexpression, while tissue inhibitor of metalloproteinases 1 (TIMP-1) expression was increased. In vivo, overexpression of SENCR improved the pathological change in aortic tissues and the damage in arterial wall elastic fibers induced by Ang-II, as well as suppressed Ang-II-induced cell apoptosis and extracellular matrix degradation. Overall, SENCR was decreased in AAA. Overexpression of SENCR inhibited AAA formation via inhibition of VSMC apoptosis and extracellular matrix degradation. We provided a reliable evidence for SENCR acting as a potential target for AAA treatment.
Downloads
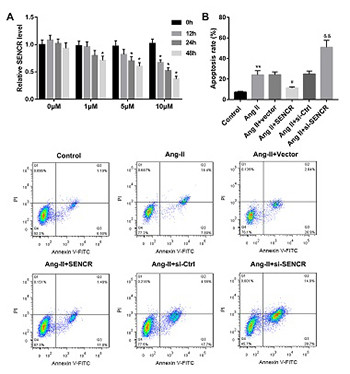