Cross-sectional imaging and cytologic investigations in the preoperative diagnosis of parotid gland tumors – An updated literature review
DOI:
https://doi.org/10.17305/bjbms.2020.5028Keywords:
Imaging, fine needle aspiration biopsy, core needle biopsy, diagnosis, parotid gland tumorsAbstract
An accurate preoperative diagnosis of parotid tumors is essential for the selection and planning of surgical treatment. Various modern cross-sectional imaging and cytologic investigations can support the differential diagnosis of parotid tumors. The aim of this study was to achieve a comprehensive and updated review of modern imaging and cytologic investigations used in parotid tumor diagnosis, based on the latest literature data. This literature review could serve as a guide for clinicians in selecting different types of investigations for the preoperative differential diagnosis of parotid tumors. Magnetic resonance imaging (MRI) with its dynamic and advanced sequences is the first-line imaging investigation used in differentiating parotid tumors. Computed tomography (CT) and positron emission tomography (PET)-CT provide limited indications in differentiating parotid tumors. Fine needle aspiration biopsy and core needle biopsy can contribute with satisfactory results to the cytological diagnosis of parotid tumors. Dynamic MRI with its dynamic contrast-enhanced and diffusion-weighted sequences provides the best accuracy for the preoperative differential diagnosis of parotid tumors. CT allows the best evaluation of bone invasion, being useful when MRI cannot be performed, and PET-CT has value in the follow-up of cancer patients. The dual cytological and imaging approach is the safest method for an accurate differential diagnosis of parotid tumors.
Citations
Downloads
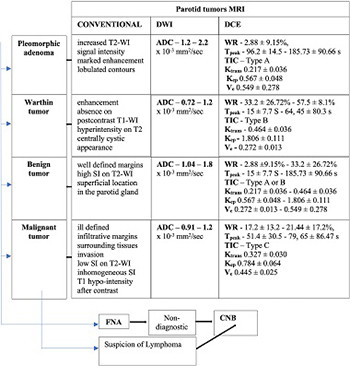
Downloads
Additional Files
Published
How to Cite
Accepted 2020-09-01
Published 2021-02-01