Epigenetically inactivated RASSF1A as a tumor biomarker
DOI:
https://doi.org/10.17305/bjbms.2020.5219Keywords:
RASSF1A, epigenetic alteration, DNA methylation, biomarkersAbstract
RASSF1A, one of the eight isoforms of the RASSF1 gene, is a tumor suppressor gene that influences tumor initiation and development. In cancer, RASSF1A is frequently inactivated by mutations, loss of heterozygosity, and, most commonly, by promoter hypermethylation. Epigenetic inactivation of RASSF1A was detected in various cancer types and led to significant interest; current research on RASSF1A promoter methylation focuses on its roles as an epigenetic tumor biomarker. Typically, researchers analyzed genomic DNA (gDNA) to measure the amount of RASSF1A promoter methylation. Cell-free DNA (cfDNA) from liquid biopsies is a recent development showing promise as an early cancer diagnostic tool using biomarkers, such as RASSF1A. This review discusses the evidence on aberrantly methylated RASSF1A in gDNA and cfDNA from different cancer types and its utility for early cancer diagnosis, prognosis, and surveillance. We compared methylation frequencies of RASSF1A in gDNA and cfDNA in various cancer types. The weaknesses and strengths of these analyses are discussed. In conclusion, although the importance of RASSSF1A methylation to cancer has been established is included in several diagnostic panels, its diagnostic utility is still experimental.
Citations
Downloads
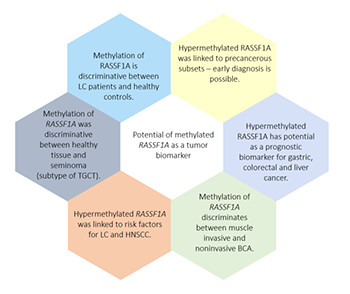
Downloads
Additional Files
Published
Issue
Section
Categories
How to Cite
Accepted 2020-11-11
Published 2021-08-01