Effects of L-dopa on expression of prolactin and synaptotagmin IV in 17-beta-estradiol-induced prolactinomas of ovariectomized hemiparkinsonian rats
DOI:
https://doi.org/10.17305/bjbms.2021.5491Keywords:
Parkinson's disease, synaptotagmin IV, L-dopa, prolactin, prolactinoma, 17-beta-estradiolAbstract
Parkinson’s disease (PD) is a long-term degenerative disorder of the central nervous system that mainly affects the motor system. Dopamine precursor levodopa (L-dopa) is used as the first-line treatment for PD. Evidence suggests neuroprotective effects of estrogens in PD. Since both 17b-estradiol (E2) and L-dopa act as regulators of prolactin (PRL) secretion from the pituitary gland, we investigated their effect on the expression of PRL in prolactinomas that developed in ovariectomized hemiparkinsonian rats treated with E2. We also investigated the effect of E2 and L-dopa on the expression of synaptotagmin IV (Syt IV), an immediate early gene whose product is abundant in the pituitary gland and was found to be highly co-expressed with PRL in lactotrophs (>90%). The hemiparkinsonian rat model was obtained by unilateral lesioning of dopaminergic nigrostriatal neurons. Rats received silastic tubing implants with E2 and were treated with L-dopa. Enzyme-linked immunosorbent assay and immunohistochemistry were used to assess the serum concentrations of PRL and E2 and expression of PRL and Syt IV in the tissue of adenohypophysis, respectively. We found that high levels of serum E2 were associated with the upregulation of Syt IV and PRL in PRL-ir cells, while treatment with L-dopa decreased the size of prolactinomas and downregulated Syt IV but had no effect on PRL expression or serum concentrations.
Citations
Downloads
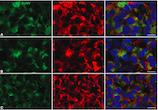
Downloads
Additional Files
Published
Issue
Section
Categories
How to Cite
Accepted 2021-03-24
Published 2021-12-01