Serum glial fibrillary acidic protein as a biomarker of brain injury in premature neonates
DOI:
https://doi.org/10.17305/bjbms.2021.6205Keywords:
Brain injury, GFAP, premature neonates, intraventricular hemorrhage, periventricular leukomalaciaAbstract
Neonatal brain injury is a serious adverse outcome of prematurity. Early detection of high risk premature neonates to develop neonatal brain injury is not currently feasible. The predictive value of many biomarkers has been tested, but none is used currently in clinical practice. The purpose of this study was to determine the levels and predictive value of serum glial fibrillary acidic protein (GFAP) in a prospective longitudinal case-control study during the first three days of life in premature neonates (<34 weeks of gestation) that later developed either intraventricular hemorrhage or periventricular leukomalacia. Each case (n=29) was matched according to birth weight and gestational age to one neonate with normal head ultrasound scans. No significant difference on GFAP levels was observed between the groups. Nevertheless, neonates with brain injury presented more frequently GFAP levels above the lowest detection limit (0.056 ng/ml) and this trend was significantly different during all days. The effectiveness of GFAP as an early biomarker of neonatal brain injury in premature neonates seems to be limited.
Citations
Downloads
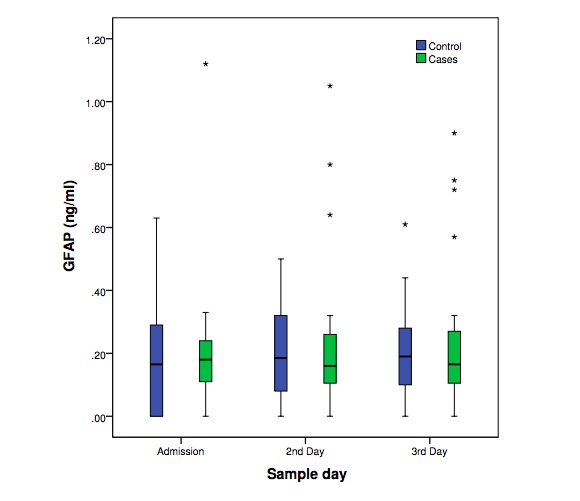
Downloads
Additional Files
Published
How to Cite
Accepted 2021-07-07
Published 2022-02-01