Sustained seroprevalence of SARS-CoV-2 antibodies one year after infection: one of the first COVID-19 cluster cases in Bosnia and Herzegovina
DOI:
https://doi.org/10.17305/bjbms.2021.6340Keywords:
Bosnia and Herzegoina, pandemic, SARS-CoV-2, COVID-19, immune response, seroconversion, IgGAbstract
SARS-CoV-2 (severe acute respiratory syndrome coronavirus type 2) is a novel virus that has been identified as a causal agent of COVID-19, an emergent infectious disease which brought about a new pandemic in the twenty-first century. The immune responses and clinical features of individuals infected with SARS-CoV-2 have not yet been fully described. Thus, in this study, we compare the seroprevalence and define the correlation between symptoms and serological results in the first COVID-19 cluster in the city of Konjic, Bosnia and Herzegovina. Of the total number, 93% of RT-PCR positive participants had positive IgG serology and 75% of them developed symptoms of COVID-19. We found that there was no significant alteration in specific IgG (p = 0.504) antibody levels during the 1-year period after COVID-19. Our results indicate that symptomatic COVID-19 patients have a higher rate of seroconversion (p < 0.01). The IgG seroconversion was correlated with high fever (p = 0.002) and headache (p = 0.007), suggesting that these symptoms could be considered as indicators of a better immune response. This study has demonstrated persistence of sustained levels of specific SARS-CoV-2 antibodies after recovering from COVID-19 infection. However, in order to gain a better insight into the immune response to SARS-CoV-2, further systematic studies should be focused on quality and longevity analyses.
Citations
Downloads
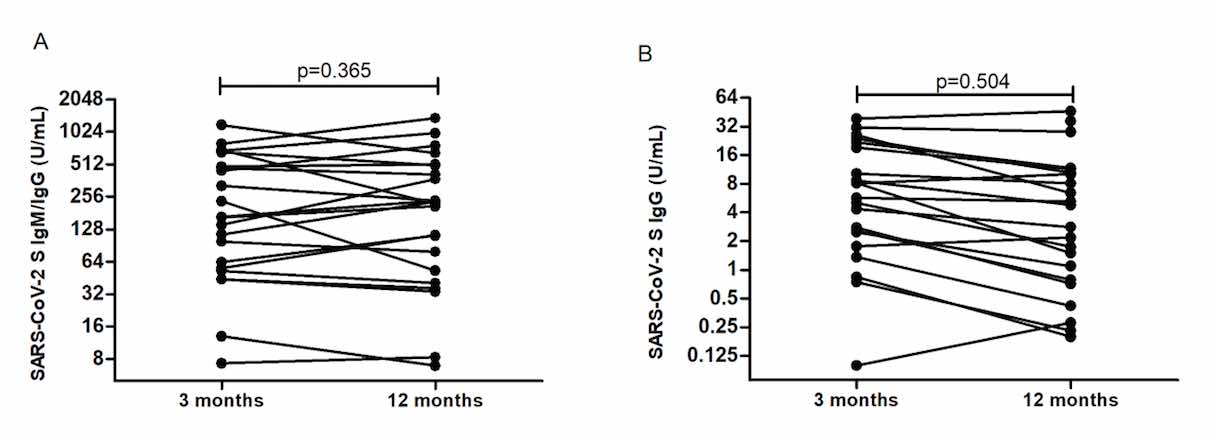
Downloads
Additional Files
Published
Issue
Section
Categories
How to Cite
Accepted 2021-08-08
Published 2022-02-01