Reactive oxygen species activated by mitochondria-specific camptothecin prodrug for enhanced chemotherapy
DOI:
https://doi.org/10.17305/bjbms.2022.7194Keywords:
Camptothecin prodrug, reactive oxygen species, antitumor therapyAbstract
Camptothecin (CPT) has attracted much attention due to its potent antitumor activities. However, the undesirable physicochemical properties, including poor water-solubility, unstable lactone ring and severe adverse effects limit its further application. In this study, two water-soluble prodrugs, CPT-lysine (CPTK) and CPT-arginine (CPTR), were designed and synthesized by conjugating lysine or arginine with CPT, improving its solubility, pharmacokinetic properties and tumor penetration. Importantly, the introduction of arginine into CPTR contributed to the mitochondria-specific delivery, which increased mitochondrial reactive oxygen species (ROS) generation, induced mitochondria dysfunction and enhanced cell apoptosis and in vivo anti-cancer effect. This strategy is believed to hold great potential for organelle-specific synergistic anti-tumor therapy.
Citations
Downloads
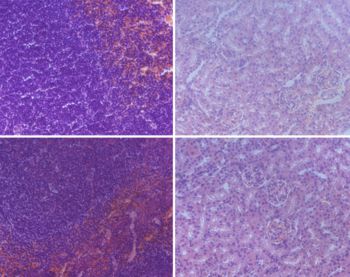
Downloads
Additional Files
Published
License
Copyright (c) 2022 Zhaopei Guo, Zian Wang, Ruifeng Liang, Huayu Tian, Xuesi Chen, Meiwan Chen

This work is licensed under a Creative Commons Attribution 4.0 International License.
How to Cite
Accepted 2022-04-14
Published 2022-10-23