Prognostic significance of HDL-C on long-term mortality in patients with COVID-19 pneumonia in the Turkish population: A potential mechanism for population differences
DOI:
https://doi.org/10.17305/bjbms.2022.7545Keywords:
COVID-19, HDL-C, lipoproteins, personalized medicine, public healthAbstract
Coronavirus disease 2019 (COVID-19) is diagnosed by the evidence of the presence of multiple phenotypes, including thrombosis, inflammation, and alveolar and myocardial damage, which can cause severe illness and mortality. High-density lipoprotein cholesterol (HDL-C) has pleiotropic properties, including anti-inflammatory, anti-infectious, antithrombotic, and endothelial cell protective effects. The aim of this study was to investigate the HDL-C levels and one-year mortality after the first wave of patients with COVID-19 were hospitalized. Data from 101 patients with COVID-19 were collected for this single-center retrospective study. Lipid parameters were collected on the admission. The relationship between lipid parameters and long-term mortality was investigated. The mean age of the non-survivor group (n = 38) was 68.8 ± 14.1 years, and 55% were male. The HDL-C levels were significantly lower in the non-survivors group compared with the survivors (26.9 ± 9.5 vs 36.8 ± 12.8 mg/dl, respectively p < 0.001). Multivariate regression analysis determined that age, C-reactive protein, D-dimer, hypertension, and HDL-C as independent predictors for the development of COVID-19 mortality. HDL-C levels <30.5 mg/dl had 71% sensitivity and 68% specificity to predict one-year mortality after COVID-19. The findings of this study showed that HDL-C is a predictor of one-year mortality in Turkish patients with COVID-19. COVID-19 is associated with decreased lipid levels, and it is an indicator of the inflammatory burden and increased mortality rate. The consequences of long-term metabolic dysregulations in patients that have recovered from COVID-19 still need to be understood.
Citations
Downloads
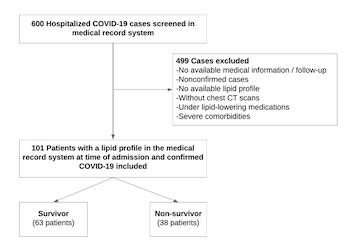
Downloads
Additional Files
Published
Issue
Section
Categories
License
Copyright (c) 2022 Ömer Faruk Baycan, Furkan Bölen, Başak Atalay, Mehmet Agirbasli

This work is licensed under a Creative Commons Attribution 4.0 International License.
How to Cite
Accepted 2022-08-20
Published 2022-10-23