C5aR1 shapes a non-inflammatory tumor microenvironment and mediates immune evasion in gastric cancer
DOI:
https://doi.org/10.17305/bjbms.2022.8317Keywords:
C5aR1, gastric cancer, immune escape, immunotherapy, tumor microenvironmentAbstract
C5a receptor 1 (C5aR1) is associated with various inflammatory processes, the pathogenesis of immune diseases, and tumor growth. However, its role in the tumor microenvironment of gastric cancer (GC) remains unclear. In this study, the expression of C5aR1 in GC and normal gastric mucosa tissues was compared using data retrieved from the Gene Expression Omnibus (GEO) and The Cancer Genome Atlas (TCGA) databases, and the results were validated by in vitro qRT-PCR and immunohistochemical analyses. The relationship between C5aR1 expression and the overall survival of patients with GC was analyzed using the Kaplan–Meier method. Subsequently, enrichment analysis was performed, and the signaling pathways were screened. C5aR1 expression was also correlated with genes related to the immune checkpoint and immune cell infiltration. The results revealed that C5aR1 expression was enhanced in GC tissues compared to normal gastric tissues, and that patients with high expression of C5aR1 had a worse 10-year overall survival compared to those showing low expression of C5aR1. Functional analysis revealed that C5aR1 is a gene related to theimmune system and may play a crucial role in inflammatory and tumor immune responses. Additionally, C5aR1 showed a positive correlation with most immune checkpoint-related genes and a negative correlation with natural killer cells, dendritic cells, and CD8+ T cells. Immune evasion risk was observed to be significantly greater in patients with higher expression of C5aR1 than in those with lower expression. The results of this study reveal that C5aR1 shapes a non-inflammatory tumor microenvironment in GC and mediates immune evasion.
Citations
Downloads
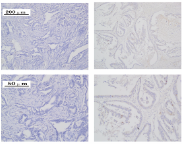
Downloads
Additional Files
Published
License
Copyright (c) 2022 Honghong Shen, Xianhua Gu, Xinwei Li, Zheng Xiang, Rong Zhang, Fan Shi, Mingyue Tang, Huiyuan Li, Guangzheng Zhu, Fang Su, Zishu Wang

This work is licensed under a Creative Commons Attribution 4.0 International License.