Identification of potential hub genes associated with atopic dermatitis-like recombinant human epidermal model using integrated transcriptomic and proteomic analysis
DOI:
https://doi.org/10.17305/bb.2023.9439Keywords:
Atopic dermatitis, transcriptomic, proteomic, recombinant human epidermal, inflammatoryAbstract
Atopic dermatitis (AD) is a severe inflammatory skin disorder, characterized by elevated levels of proinflammatory cytokines that fuel a vicious cycle of inflammation. While inflammatory recombinant human epidermal (RHE) models relevant to AD have been established, comprehensive understanding remains limited. To illuminate changes and identify potential hub genes involved in AD-related inflammation, RHE models, stimulated by an inflammatory cocktail including polyinosinic-polycytidylic acid, tumor necrosis factor alpha (TNF-α), interleukin 4 (IL-4) and interleukin 13 (IL-13), were constructed and examined using tandem mass tags-proteomic coupled with RNA-seq transcriptomic analyses. Principal component analysis (PCA), Gene Ontology (GO), and Kyoto Encyclopedia of Genes and Genomes (KEGG) pathway functional enrichment were employed for the analysis of related genes and proteins. Protein-protein interaction networks helped identify hub genes, which were further confirmed by qPCR and western blot. We observed high expression of thymic stromal lymphopoietin in the inflammatory RHE. Our study identified 2369 differentially expressed genes and 880 differentially expressed proteins in the cocktail-induced group versus the normal control group. A total of 248 overlapping symbols were enriched in various biological processes and signaling pathways, including cornification envelope, cell-cell junction, calcium ion binding, extracellular matrix receptor, terpenoid backbone biosynthesis, and peroxisome proliferator-activated receptors signaling pathway, among others. Among the 248 overlapping symbols, CytoHubba identified 10 hub molecules, namely signal transducer and activator of transcription 3 (STAT3), integrin subunit beta 1 (ITGB1), filaggrin (FLG), involucrin (IVL), DEAD (Asp-Glu-Ala-Asp) box polypeptide 58 (DDX58), small proline rich protein 1B (SPRR1B), interferon induced with helicase C domain 1 (IFIH1), desmoglein 1 (DSG1), collagen type XVII alpha 1 chain (COL17A1), and integrin subunit alpha 6 (ITGA6), based on the degree. These integrated results offer valuable insights into the molecular mechanisms of AD and present potential tools for screening cosmetic formulations intended for the treatment of AD.
Citations
Downloads
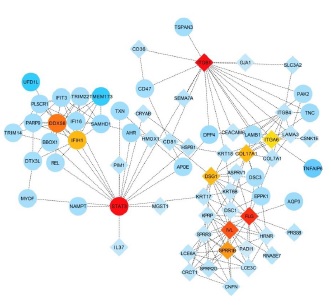
Downloads
Additional Files
Published
License
Copyright (c) 2023 Wu Qiao, Tong Xie, Jing Lu, Tinghan Jia, Ken Kaku

This work is licensed under a Creative Commons Attribution 4.0 International License.
How to Cite
Accepted 2023-07-19
Published 2024-01-03