Hypolipidemic and antioxidant effects of buckwheat leaf and flower mixture in hyperlipidemic rats
DOI:
https://doi.org/10.17305/bjbms.2013.2389Keywords:
buckwheat, plasma lipids, plasma phospholipids fatty acids, oxidative stress, hypolipidemic effectAbstract
As a source of biologically active compounds, buckwheat has beneficial effects in nutrition due to its high content of flavonoids, particularly rutin. Aim of our study was to examine effects of buckwheat on plasma lipid status and phospholipids fatty acids composition, histological and parameters of oxidative stress in Wistar rats fed a high-fat diet. This study showed that buckwheat leaf and flower (BLF) mixture supplementation significantly reduce weight gain, plasma lipid concentrations and atherogenic index in rats fed a high-fat diet. Treatment of the high-fat group of animals with buckwheat significantly increased percentage of n-6 fatty acids as well as eicosapentaenoic acid (EPA) and decreased percentage of saturated fatty acids (SFA) and oleic acid. Buckwheat antioxidant effects diminished negative influence of high-fat diet in hyperlipidemic rats, while pathohistological analysis of liver confirmed changes after high-fat consumption. Our results showed hypolipidemic, antiatherogenic and antioxidative features of buckwheat leaf and flower mixture, and these parts of the plant with the highest rutin content could be beneficial in prevention and curing of hyperlipidemia.
Citations
Downloads
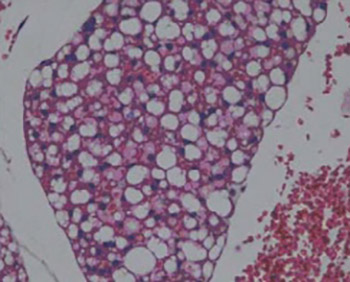
Downloads
Additional Files
Published
How to Cite
Accepted 2017-08-30
Published 2013-05-20