Dexmedetomidine reduces ventilator-induced lung injury (VILI) by inhibiting Toll-like receptor 4 (TLR4)/nuclear factor (NF)-κB signaling pathway
DOI:
https://doi.org/10.17305/bjbms.2018.2400Keywords:
Dexmedetomidine, ventilator-induced lung injury, VILI, Toll-like receptor 4, TLR4, Nuclear factor κB, NF-κB, inflammatory cytokine, α2-adrenoceptorAbstract
Mechanical ventilation (MV) may lead to ventilator-induced lung injury (VILI). Previous research has shown that dexmedetomidine attenuates pulmonary inflammation caused by MV, but the underlying mechanisms remain unclear. Our study aims to test whether dexmedetomidine has a protective effect against VILI and to explore the possible molecular mechanisms using the rat model. Thirty adult male Wistar rats weighing 200-250 g were randomly assigned to 5 groups (n = 6): control, low tidal volume MV (LMV), high tidal volume (HVT) MV (HMV), HVT MV + dexmedetomidine (DEX), HVT MV + dexmedetomidine + yohimbine (DEX+Y). Rats were euthanized after being ventilated for 4 hours. Pathological changes, lung wet/dry (W/D) weight ratio, lung myeloperoxidase (MPO) activity, levels of inflammatory cytokines (i.e., interleukin [IL]-1β, tumor necrosis factor alpha [TNF-α], and IL-6) in the bronchoalveolar lavage fluid (BALF) and lung tissues, expression of Toll-like receptor 4 (TLR4) and nuclear factor (NF)-κB, and activation of NF-κB in lung tissues were measured. Compared with HMV, DEX group showed fewer pathological changes, lower W/D ratios and decreased MPO activity of the lung tissues and lower concentrations of the inflammatory cytokines in the BALF and lung tissues. Dexmedetomidine significantly inhibited the expression of TLR4 and NF-κB and activation of NF-κB. Yohimbine partly alleviated the effects of dexmedetomidine. Dexmedetomidine reduced the inflammatory response to HVT-MV and had a protective effect against VILI, with the inhibition of the TLR4/NF-κB signaling pathway, at least partly via α2-adrenoceptors.
Citations
Downloads
References
Petrucci N, De Feo C. Lung protective ventilation strategy for the acute respiratory distress syndrome. Cochrane Database Syst Rev 2013;(2):CD003844. https://doi.org/10.1002/14651858.CD003844.pub4.
Loring SH, Talmor D. Inhomogeneous computed tomographic densities in lungs in acute respiratory distress syndrome: Stress multipliers leading to ventilator-induced injury? Am J Respir Crit Care Med 2014;189(2):123-4.
https://doi.org/10.1164/rccm.201312-2189ED2189ED.
Slutsky AS, Ranieri VM. Ventilator-induced lung injury. N Engl J Med 2013;369(22):2126-36. https://doi.org/10.1056/NEJMra1208707.
Scheiermann J, Klinman DM. Suppressive oligonucleotides inhibit inflammation in a murine model of mechanical ventilator induced lung injury. J Thorac Dis 2016;8(9):2434-43. https://doi.org/10.21037/jtd.2016.08.18.
Ko YA, Yang MC, Huang HT, Hsu CM, Chen LW. NF-κB activation in myeloid cells mediates ventilator-induced lung injury. Respir Res 2013;14(1):69. https://doi.org/10.1186/1465-9921-14-69.
Cruickshank M, Henderson L, MacLennan G, Fraser C, Campbell M, Blackwood B, et al. Alpha-2 agonists for sedation of mechanically ventilated adults in intensive care units: A systematic review. Health Technol Assess 2016;20(25):v-xx, 1-117. https://doi.org/10.3310/hta20250.
Li B, Li Y, Tian S, Wang H, Wu H, Zhang A, et al. Anti-inflammatory effects of perioperative dexmedetomidine administered as an adjunct to general anesthesia: A meta-analysis. Sci Rep 2015;5:12342. https://doi.org/10.1038/srep12342.
Schoeler M, Loetscher PD, Rossaint R, Fahlenkamp AV, Eberhardt G, Rex S, et al. Dexmedetomidine is neuroprotective in an in vitro model for traumatic brain injury. BMC Neurol 2012;12:20. https://doi.org/10.1186/1471-2377-12-20.
Chen C, Zhang Z, Chen K, Zhang F, Peng M, Wang Y. Dexmedetomidine regulates inflammatory molecules contributing to ventilator-induced lung injury in dogs. J Surg Res 2014;187(1):211-8. https://doi.org/10.1016/j.jss.2013.09.018.
Medzhitov R, Janeway C Jr. Innate immune recognition: Mechanisms and pathways. Immunol Rev 2000;173:89-97. https://doi.org/10.1034/j.1600-065X.2000.917309.x.
Takeda K, Akira S. Toll receptors and pathogen resistance. Cell Microbiol 2003;5(3):143-53. https://doi.org/10.1046/j.1462-5822.2003.00264.x.
Takeuchi O, Hoshino K, Kawai T, Sanjo H, Takada H, Ogawa T, et al. Differential roles of TLR2 and TLR4 in recognition of gram-negative and gram-positive bacterial cell wall components. Immunity 1999;11(4):443-51. https://doi.org/10.1016/S1074-7613(00)80119-3.
Guijarro-Munoz I, Compte M, Alvarez-Cienfuegos A, Alvarez-Vallina L, Sanz L. Lipopolysaccharide activates Toll-like receptor 4 (TLR4)-mediated NF-κB signaling pathway and proinflammatory response in human pericytes. J Biol Chem 2014;289(4):2457-68. https://doi.org/10.1074/jbc.M113.521161.
Wong ET, Tergaonkar V. Roles of NF-κB in health and disease: Mechanisms and therapeutic potential. Clin Sci (Lond) 2009;116(6):451-65. https://doi.org/10.1042/CS20080502.
Hu G, Malik AB, Minshall RD. Toll-like receptor 4 mediates neutrophil sequestration and lung injury induced by endotoxin and hyperinflation. Crit Care Med 2010;38(1):194-201. https://doi.org/10.1097/CCM.0b013e3181bc7c17.
Lucas K, Maes M. Role of the Toll Like receptor (TLR) radical cycle in chronic inflammation: Possible treatments targeting the TLR4 pathway. Mol Neurobiol 2013;48(1):190-204. https://doi.org/10.1007/s12035-013-8425-7.
Peri F, Calabrese V. Toll-like receptor 4 (TLR4) modulation by synthetic and natural compounds: An update. J Med Chem 2014;57(9):3612-22. https://doi.org/10.1021/jm401006s.
Li H, Su X, Yan X, Wasserloos K, Chao W, Kaynar AM, et al. Toll-like receptor 4-myeloid differentiation factor 88 signaling contributes to ventilator-induced lung injury in mice. Anesthesiology 2010;113(3):619-29. https://doi.org/10.1097/ALN.0b013e3181e89ab2.
Villar J, Cabrera NE, Casula M, Flores C, Valladares F, Diaz-Flores L, et al. Mechanical ventilation modulates TLR4 and IRAK-3 in a non-infectious, ventilator-induced lung injury model. Respir Res 2010;11:27. https://doi.org/10.1186/1465-9921-11-27.
Vaneker M, Joosten LA, Heunks LM, Snijdelaar DG, Halbertsma FJ, van Egmond J, et al. Low-tidal-volume mechanical ventilation induces a toll-like receptor 4-dependent inflammatory response in healthy mice. Anesthesiology 2008;109(3):465-72. https://doi.org/10.1097/ALN.0b013e318182aef1.
Gajic O, Dara SI, Mendez JL, Adesanya AO, Festic E, Caples SM, et al. Ventilator-associated lung injury in patients without acute lung injury at the onset of mechanical ventilation. Crit Care Med 2004;32(9):1817-24. https://doi.org/10.1097/01.CCM.0000133019.52531.30.
Dhanireddy S, Altemeier WA, Matute-Bello G, O'Mahony DS, Glenny RW, Martin TR, et al. Mechanical ventilation induces inflammation, lung injury, and extra-pulmonary organ dysfunction in experimental pneumonia. Lab Invest 2006;86(8):790-9. https://doi.org/10.1038/labinvest.3700440.
Sen V, Guzel A, Sen HS, Ece A, Uluca U, Soker S, et al. Preventive effects of dexmedetomidine on the liver in a rat model of acid-induced acute lung injury. Biomed Res Int 2014;2014:621827. https://doi.org/10.1155/2014/621827.
Chen Y, Miao L, Yao Y, Wu W, Wu X, Gong C, et al. Dexmedetomidine ameliorate CLP-induced rat intestinal injury via inhibition of inflammation. Mediators Inflamm 2015;2015:918361. https://doi.org/10.1155/2015/918361.
Hayden MS, Ghosh S. NF-κB in immunobiology. Cell Res 2011;21(2):223-44. https://doi.org/10.1038/cr.2011.13.
Hayden MS, Ghosh S. Regulation of NF-κB by TNF family cytokines. Semin Immunol 2014;26(3):253-66. https://doi.org/10.1016/j.smim.2014.05.004.
Hayden MS, Ghosh S. NF-κB, the first quarter-century: Remarkable progress and outstanding questions. Genes Dev 2012;26(3):203-34. https://doi.org/10.1101/gad.183434.111.
Ghosh G, Wang VY, Huang DB, Fusco A. NF-κB regulation: Lessons from structures. Immunol Rev 2012;246(1):36-58. https://doi.org/10.1111/j.1600-065X.2012.01097.x.
Hoesel B, Schmid JA. The complexity of NF-κB signaling in inflammation and cancer. Mol Cancer 2013;12:86. https://doi.org/10.1186/1476-4598-12-86.
Camp SM, Ceco E, Evenoski CL, Danilov SM, Zhou T, Chiang ET, et al. Unique Toll-like receptor 4 activation by NAMPT/PBEF induces NFκB signaling and inflammatory lung injury. Sci Rep 2015;5:13135. https://doi.org/10.1038/srep13135.
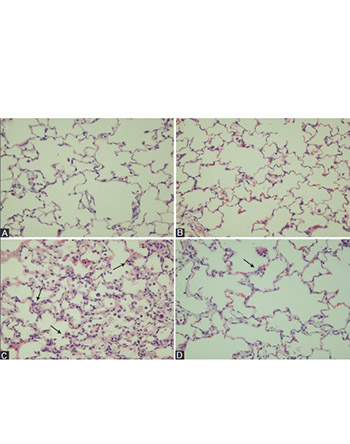
Downloads
Additional Files
Published
How to Cite
Accepted 2017-11-14
Published 2018-05-20