Glycogen accumulation in cardiomyocytes and cardiotoxic effects after 3NPA treatment.
DOI:
https://doi.org/10.17305/bjbms.2012.2525Keywords:
rat, heart, 3NPA, toxicity, glycogenAbstract
Mitochondrial toxin 3-nitropropionic acid (3NPA) is a neurotoxin that inhibits the activity of succinate dehydrogenase, a key enzyme of oxidative energy production, and characteristically provokes neurodegeneration in the striatum, resembling Huntington’s disease. 3NPA also affects the activity of glycogen-sinthase-kinase-3b (GSK-3b), an enzyme implicated in glycogen synthesis and in signal transduction. The aim of this study was to evaluate cardiac glycogen content and histopathological changes in the hearts of rats after subchronic treatment with 3NPA. Female adult Wistar rats were treated daily with 30mg/kg of 3NPA subcutaneously 8 days. The control group was treated with normal saline for 8 days. For the comparison of measured parameters between groups we used the Student’s t-test (p<0.05).
The stereological evaluation of glycogen content in histological sections of the heart was processed with periodic acid-Schiff (PAS). Histochemical procedure showed a significant accumulation of glycogen granules in the 3NPA group (0.028mm3/mm3±0.022), whereas the hearts of control animals were nearly devoid of glycogen granules (0.002mm3/mm3±0.001). Haematoxylin-eosin histological staining showed diffuse swelling of cardiomyocytes (3NPA=15.989μm ±1.649; saline=13.456μm ± 0.786), loss of cell cross-striations, lower myofibril volume fraction (3NPA=0.3922mm3/mm3 ± 0.0230, saline=0.4ssomm3/mm3 ± 0.0083), and mononuclear infiltration in the interstitial tissue, mostly along the blood vessels. Sirius red staining showed fibrosis of the heart (3NPA=0.0531mm3/mm3±0.0090, saline=0.013smm3/mm3 ± 0.0051). TUNEL staining showed TUNEL-positive cells in the 3NPA group (2.04cells/mm2 ± 0.92) and almost no TUNEL-positive cells in the saline group (027cells/mm2 ± 0.14).
This experiment shows that 3NPA-induced histopathological changes in the heart are accompanied by a significant accumulation of glycogen granules in cardiomyocytes.
Citations
Downloads
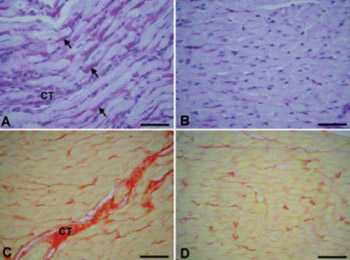
Downloads
Additional Files
Published
Issue
Section
Categories
How to Cite
Accepted 2017-10-02
Published 2012-02-20