Modulatory effect of curcumin on ketamine-induced toxicity in rat thymocytes: Involvement of reactive oxygen species (ROS) and the phosphoinositide 3-kinase (PI3K)/protein kinase B (Akt) pathway
DOI:
https://doi.org/10.17305/bjbms.2018.2607Keywords:
Ketamine, curcumin, toxicity, thymocytes, PI3K/Akt signaling pathway, anti-apoptotic effect, protective effect, apoptosis, reactive oxygen speciesAbstract
Ketamine is a widely used anesthetic in pediatric clinical practice. Previous studies have demonstrated that ketamine induces neurotoxicity and has a modulatory effect on the cells of the immune system. Here, we evaluated the potential protective effect and underlying mechanisms of natural phenolic compound curcumin against ketamine-induced toxicity in rat thymocytes. Rat thymocytes were exposed to 100 µM ketamine alone or combined with increasing concentrations of curcumin (0.3, 1, and 3 μM) for 24 hours. Cell viability was analyzed with CCK-8 assay kit. Apoptosis was analyzed using flow cytometry and propidium iodide as well as Z-VAD-FMK and Z-LEHD-FMK inhibitors. Reactive oxygen species (ROS) production and mitochondrial membrane potential [MMP] were measured by flow cytometry. Colorimetric assay with DEVD-pNA substrate was used for assessing caspase-3 activity. Involvement of phosphoinositide 3-kinase (PI3K)/protein kinase B (Akt) signaling pathway was tested with Wortmannin inhibitor. Ketamine induced toxicity in cells, increased the number of hypodiploid cells, caspase-3 activity and ROS production, and inhibited the MMP. Co-incubation of higher concentrations of curcumin (1 and 3 μM) with ketamine markedly decreased cytotoxicity, apoptosis rate, caspase-3 activity, and ROS production in rat thymocytes, and increased the MMP. Application of Z-VAD-FMK (a pan caspase inhibitor) or Z-LEHD-FMK (caspase-9 inhibitor) with ketamine effectively attenuated the ketamine-induced apoptosis in rat thymocytes. Administration of Wortmannin (a PI3K inhibitor) with curcumin and ketamine significantly decreased the protective effect of curcumin on rat thymocytes. Our results indicate that ketamine-induced toxicity in rat thymocytes mainly occurs through the mitochondria-mediated apoptotic pathway and that the PI3K/Akt signaling pathway is involved in the anti-apoptotic effect of curcumin.
Citations
Downloads
References
Bai X, Yan Y, Canfield S, Muravyeva MY, Kikuchi C, Zaja I, et al. Ketamine enhances human neural stem cell proliferation and induces neuronal apoptosis via reactive oxygen species-mediated mitochondrial pathway. Anesth Analg 2013;116(4):869-80. https://doi.org/10.1213/ANE.0b013e3182860fc9.
Slikker W Jr, Zou X, Hotchkiss CE, Divine RL, Sadovova N, Twaddle NC, et al. Ketamine-induced neuronal cell death in the perinatal rhesus monkey. Toxicol Sci 2007;98(1):145-58. https://doi.org/10.1093/toxsci/kfm084.
Ikonomidou C, Bosch F, Miksa M, Bittigau P, Vockler J, Dikranian K, et al. Blockade of NMDA receptors and apoptotic neurodegeneration in the developing brain. Science 1999;283(5398):70-4. https://doi.org/10.1126/science.283.5398.70.
Soriano SG, Liu Q, Li J, Liu JR, Han XH, Kanter JL, et al. Ketamine activates cell cycle signaling and apoptosis in the neonatal rat brain. Anesthesiology 2010;112(5):1155-63. https://doi.org/10.1097/ALN.0b013e3181d3e0c2.
Li J, Wu H, Xue G, Wang P, Hou Y. 17beta-oestradiol protects primary-cultured rat cortical neurons from ketamine-induced apoptosis by activating PI3K/Akt/Bcl-2 signalling. Basic Clin Pharmacol Toxicol 2013;113(6):411-8. https://doi.org/10.1111/bcpt.12124.
Braun S, Gaza N, Werdehausen R, Hermanns H, Bauer I, Durieux ME, et al. Ketamine induces apoptosis via the mitochondrial pathway in human lymphocytes and neuronal cells. Br J Anaesth 2010;105(3):347-54. https://doi.org/10.1093/bja/aeq169.
Zuo D, Lin L, Liu Y, Wang C, Xu J, Sun F, et al. Baicalin attenuates ketamine-induced neurotoxicity in the developing rats: Involvement of PI3K/Akt and CREB/BDNF/Bcl-2 pathways. Neurotox Res 2016;30(2):159-72. https://doi.org/10.1007/s12640-016-9611-y.
Aggarwal BB, Harikumar KB. Potential therapeutic effects of curcumin, the anti-inflammatory agent, against neurodegenerative, cardiovascular, pulmonary, metabolic, autoimmune and neoplastic diseases. Int J Biochem Cell Biol 2009;41(1):40-59. https://doi.org/10.1016/j.biocel.2008.06.010.
Chan WH, Wu CC, Yu JS. Curcumin inhibits UV irradiation-induced oxidative stress and apoptotic biochemical changes in human epidermoid carcinoma A431 cells. J Cell Biochem 2003;90(2):327-38. https://doi.org/10.1002/jcb.10638.
Fu Y, Zheng S, Lin J, Ryerse J, Chen A. Curcumin protects the rat liver from CCl4-caused injury and fibrogenesis by attenuating oxidative stress and suppressing inflammation. Mol Pharmacol 2008;73(2):399-409. https://doi.org/10.1124/mol.107.039818.
Woo JM, Shin DY, Lee SJ, Joe Y, Zheng M, Yim JH, et al. Curcumin protects retinal pigment epithelial cells against oxidative stress via induction of heme oxygenase-1 expression and reduction of reactive oxygen. Mol Vis 2012;18:901-8.
Jagetia GC, Aggarwal BB. "Spicing up" of the immune system by curcumin. J Clin Immunol 2007;27(1):19-35. https://doi.org/10.1007/s10875-006-9066-7.
Varalakshmi CH, Ali AM, Pardhasaradhi BV, Srivastava RM, Singh S, Khar A. Immunomodulatory effects of curcumin: In-vivo. Int Immunopharmacol 2008;8(5):688-700. https://doi.org/10.1016/j.intimp.2008.01.008.
Seyedzadeh MH, Safari Z, Zare A, Gholizadeh Navashenaq J, Razavi SA, Kardar GA, et al. Study of curcumin immunomodulatory effects on reactive astrocyte cell function. Int Immunopharmacol 2014;22(1):230-5. https://doi.org/10.1016/j.intimp.2014.06.035.
Pavlovic V, Cekic S, Kocic G, Sokolovic D, Zivkovic V. Effect of monosodium glutamate on apoptosis and Bcl-2/Bax protein level in rat thymocyte culture. Physiol Res 2007;56(5):619-26.
Pavlovic V, Cekic S, Ciric M, Krtinic D, Jovanovic J. Curcumin attenuates Mancozeb-induced toxicity in rat thymocytes through mitochondrial survival pathway. Food Chem Toxicol 2016;88:105-11. https://doi.org/10.1016/j.fct.2015.12.029.
Lindgren A, Pavlovic V, Flach CF, Sjöling A, Lundin S. Interferon-gamma secretion is induced in IL-12 stimulated human NK cells by recognition of Helicobacter pylori or TLR2 ligands. Innate Immun 2011;17(2):191-203. https://doi.org/10.1177/1753425909357970.
Nishimura Y, Oyama TB, Sakanashi Y, Oyama TM, Matsui H, Okano Y, et al. Some characteristics of quercetin-induced cytotoxicity on rat thymocytes under in vitro condition. Toxicol In Vitro 2008;22(4):1002-7. https://doi.org/10.1016/j.tiv.2008.02.006.
Park GB, Choi Y, Kim YS, Lee HK, Kim D, Hur DY. ROS and ERK1/2-mediated caspase-9 activation increases XAF1 expression in dexamethasone-induced apoptosis of EBV-transformed B cells. Int J Oncol 2013;43(1):29-38. https://doi.org/10.3892/ijo.2013.1949.
Ito H, Uchida T, Makita K. Ketamine causes mitochondrial dysfunction in human induced pluripotent stem cell-derived neurons. Plos One 2015;10(5):e0128445. https://doi.org/10.1371/journal.pone.0128445.
Dallimore D, Herd DW, Short T, Anderson BJ. Dosing ketamine for pediatric procedural sedation in the emergency department. Pediatr Emerg Care 2008;24(8):529-33. https://doi.org/10.1097/PEC.0b013e318180fdb5.
Zeng J, Xia S, Zhong W, Li J, Lin L. In vitro and in vivo effects of ketamine on generation and function of dendritic cells. J Pharmacol Sci 2011;117(3):170-9. https://doi.org/10.1254/jphs.11113FP.
Pavlovic V, Cekic S, Kamenov B, Ciric M, Krtinic D. The effect of ascorbic acid on Mancozeb induced toxicity in rat thymocytes. Fol Biol (Praha) 2015;61(3):116-23.
Koizumi K, Kawanai T, Hashimoto E, Kanbara Y, Masuda T, Kanemaru K, et al. Cytometric analysis on cytotoxicity of curcumin on rat thymocytes: Proapoptotic and antiapoptotic actions of curcumin. Toxicol In Vitro 2011;25(4):985-90. https://doi.org/10.1016/j.tiv.2011.03.010.
Hori R, Kashiba M, Toma T, Yachie A, Goda N, Makino N, et al. Gene transfection of H25A mutant heme oxygenase-1 protects cells against hydroperoxide-induced cytotoxicity. J Biol Chem 2002;277:10712-8. https://doi.org/10.1074/jbc.M107749200.
Pavlovic V, Stojanovic I, Jadranin M, Vajs V, Djordjevic I, Smelcerovic A, et al. Effect of four lichen acids isolated from Hypogymnia physodes on viability of rat thymocytes. Food Chem Toxicol 2013;51:160-4. https://doi.org/10.1016/j.fct.2012.04.043.
Nicoletti I, Migliorati G, Pagliacci MC, Grignani F, Riccardi C. A rapid and simple method for measuring thymocyte apoptosis by propidium iodide staining and flow cytometry. J Immunol Methods 1991;139(2):271-9. https://doi.org/10.1016/0022-1759(91)90198-O.
Wang ZB, Liu YQ, Zhang Y, Li Y, An XX, Xu H, et al. Reactive oxygen species, but not mitochondrial membrane potential, is associated with radiation-induced apoptosis of AHH-1 human lymphoblastoid cells. Cell Biol Int 2007;31(11):1353-8. https://doi.org/10.1016/j.cellbi.2007.05.009.
Singh MK, Yadav SS, Gupta V, Khattri S. Immunomodulatory role of Emblica officinalis in arsenic induced oxidative damage and apoptosis in thymocytes of mice. BMC Complement Altern Med 2013;13:193. https://doi.org/10.1186/1472-6882-13-193.
Das A, Hazra TK, Boldogh I, Mitra S, Bhakat KK. Induction of the human oxidized base-specific DNA glycosylase Neil1 by reactive oxygen species. J Biol Chem 2005;280:35272-80. https://doi.org/10.1074/jbc.M505526200.
Boldogh I, Roy G, Lee MS, Bacsi A, Hazra TK, Bhakat KK, et al. Reduced DNA double strand breaks in chlorambucil resistant cells are related to high DNA-PKCs activity and low oxidative stress. Toxicology 2003;193(1-2):137-52. https://doi.org/10.1016/j.tox.2003.08.013.
Kang N, Zhang JH, Qiu F, Tashiro S, Onodera S, Ikejima T. Inhibition of EGFR signaling augments oridonin-induced apoptosis in human laryngeal cancer cells via enhancing oxidative stress coincident with activation of both the intrinsic and extrinsic apoptotic pathways. Cancer Lett 2010;294(2):147-58. https://doi.org/10.1016/j.canlet.2010.01.032.
Elmore S. Apoptosis: A review of programmed cell death. Toxicol Pathol 2007;35(4):495-516. https://doi.org/10.1080/01926230701320337.
Green DR, Kroemer G. The pathophysiology of mitochondrial cell death. Science 2004;305(5684):626-9. https://doi.org/10.1126/science.1099320.
Fulda S. Modulation of mitochondrial apoptosis by PI3K inhibitors. Mitochondrion 2013;13(3):195-8. https://doi.org/10.1016/j.mito.2012.05.001.
Kang N, Wang MM, Wang YH, Zhang ZN, Cao HR, Lv YH, et al. Tetrahydrocurcumin induces G2/M cell cycle arrest and apoptosis involving p38 MAPK activation in human breast cancer cells. Food Chem Toxicol 2014;67:193-200. https://doi.org/10.1016/j.fct.2014.02.024.
Fu XY, Yang MF, Cao MZ, Li DW, Yang XY, Sun JY, et al. Strategy to suppress oxidative damage-induced neurotoxicity in PC12 cells by curcumin: The role of ROS-mediated DNA damage and the MAPK and AKT pathways. Mol Neurobiol 2016;53(1):369-78. https://doi.org/10.1007/s12035-014-9021-1.
Chang Y, Chen TL, Sheu JR, Chen RM. Suppressive effects of ketamine on macrophage functions. Toxicol Appl Pharmacol 2005;204(1):27-35. https://doi.org/10.1016/j.taap.2004.08.011.
Pelicano H, Carney D, Huang P. ROS stress in cancer cells and therapeutic implications. Drug Resist Updat 2004;7(2):97-110. https://doi.org/10.1016/j.drup.2004.01.004.
Bosnjak ZJ, Yan Y, Canfield S, Muravyeva MY, Kikuchi C, Wells CW, et al. Ketamine induces toxicity in human neurons differentiated from embryonic stem cells via mitochondrial apoptosis pathway. Curr Drug Saf 2012;7(2):106-19. https://doi.org/10.2174/157488612802715663.
Victor VM, Guayerbas N, De IF. Changes in the antioxidant content of mononuclear leukocytes from mice with endotoxin-induced oxidative stress. Mol Cell Biochem 2002;229(1-2):107-11. https://doi.org/10.1023/A:1017976629018.
Hildeman DA, Mitchell T, Aronow B, Wojciechowski S, Kappler J, Marrack P. Control of Bcl-2 expression by reactive oxygen species. Proc Natl Acad Sci USA 2003;100(25):15035-40. https://doi.org/10.1073/pnas.1936213100.
Sebastia N, Montoro A, Montoro A, Almonacid M, Villaescusa JI, Cervera J, et al. Assessment in vitro of radioprotective efficacy of curcumin and resveratrol. Radiat Meas 2011;46(9):962-6. https://doi.org/10.1016/j.radmeas.2011.05.009.
Shafaghati N, Hedayati M, Hosseinimehr SJ. Protective effects of curcumin against genotoxicity induced by 131-iodine in human cultured lymphocyte cells. Pharmacogn Mag 2014;10(38):106-10. https://doi.org/10.4103/0973-1296.131020.
Qin XY, Lv JH, Cui J, Fang X, Zhang Y. Curcumin protects against staurosporine toxicity in rat neurons. Neurosci Bull 2012;28(5):606-10. https://doi.org/10.1007/s12264-012-1275-x.
Waseem M, Parvez S. Mitochondrial dysfunction mediated cisplatin induced toxicity: Modulatory role of curcumin. Food Chem Toxicol 2013;53:334-42. https://doi.org/10.1016/j.fct.2012.11.055.
Zhong F, Yang J, Tong ZT, Chen LL, Fan LL, Wang F, et al. Guggulsterone inhibits human cholangiocarcinoma Sk-ChA-1 and Mz-ChA-1 cell growth by inducing caspase-dependent apoptosis and downregulation of survivin and Bcl-2 expression. Oncol Lett 2015;10(3):1416-22. https://doi.org/10.3892/ol.2015.3391.
Hao F, Kang J, Cao Y, Fan S, Yang H, An Y, et al. Curcumin attenuates palmitate-induced apoptosis in MIN6 pancreatic β-cells through PI3K/Akt/FoxO1 and mitochondrial survival pathways. Apoptosis 2015;20(11):1420-32. https://doi.org/10.1007/s10495-015-1150-0.
Dai C, Li D, Gong L, Xiao X, Tang S. Curcumin ameliorates furazolidone-induced DNA damage and apoptosis in human hepatocyte L02 cells by inhibiting ROS production and mitochondrial pathway. Molecules 2016;21(8). PII: E1061. https://doi.org/10.3390/molecules21081061.
Jiang X, Tang X, Zhang P, Liu G, Guo H. Cyanidin-3-O-beta-glucoside protects primary mouse hepatocytes against high glucose-induced apoptosis by modulating mitochondrial dysfunction and the PI3K/Akt pathway. Biochem Pharmacol 2014;90(2):135-44. https://doi.org/10.1016/j.bcp.2014.04.018.
Li WX, Chen SF, Chen LP, Yang GY, Li JT, Liu HZ, et al. Thimerosal-induced apoptosis in mouse C2C12 myoblast cells occurs through suppression of the PI3K/Akt/survivin pathway. PloS One 2012;7(11):e49064. https://doi.org/10.1371/journal.pone.0049064.
Atif F, Yousuf S, Stein DG. Anti-tumor effects of progesterone in human glioblastoma multiforme: Role of PI3K/Akt/mTOR signaling. J Steroid Biochem Mol Biol 2015;146:62-73. https://doi.org/10.1016/j.jsbmb.2014.04.007.
Yu W, Zha W, Ke Z, Min Q, Li C, Sun H, et al. Curcumin protects neonatal rat cardiomyocytes against high glucose-induced apoptosis via PI3K/Akt signalling pathway. J Diabetes Res 2016;2016:4158591. https://doi.org/10.1155/2016/4158591.
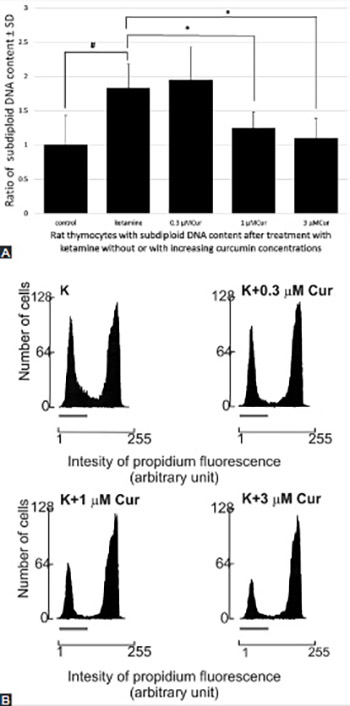