Emerging roles of sortilin in affecting the metabolism of glucose and lipid profiles
DOI:
https://doi.org/10.17305/bjbms.2021.6601Keywords:
Sortilin, dyslipidemia, insulin resistance, diabetes melitus, cardiovascular diseaseAbstract
Dyslipidemia has recently been identified as an important factor in modulating the progression of several health conditions, grouped as cardio-metabolic syndrome and including obesity,insulin resistance, and atherosclerosis. Among multiple factors which regulate the development of cardio-metabolic syndrome, sortilin has been found in multiple cell types, such as adipocyte, hepatocyte, and macrophage, suggesting that sortilin is correlated to the development and the severity of cardio-metabolic syndrome. Consistently, several genome-wide association (GWAS) and basic experimental research studies are being conducted to find novel gene loci involved in regulating the pathological progression of cardio-metabolic syndrome. According to these data, both SORT1 gene and sortilin protein have an important function in regulating the circulating lipid and glucose metabolism resulting in modulation of disease progression. In this comprehensive review, we summarize the recent research results in regards to sortilin function in modulating the circulating lipid and glucose metabolism. Moreover, we also discuss and analyze the emerging evidence elucidating the potential mechanisms by which sortilin affects synthesis and secretion of lipid and glucose.
Citations
Downloads
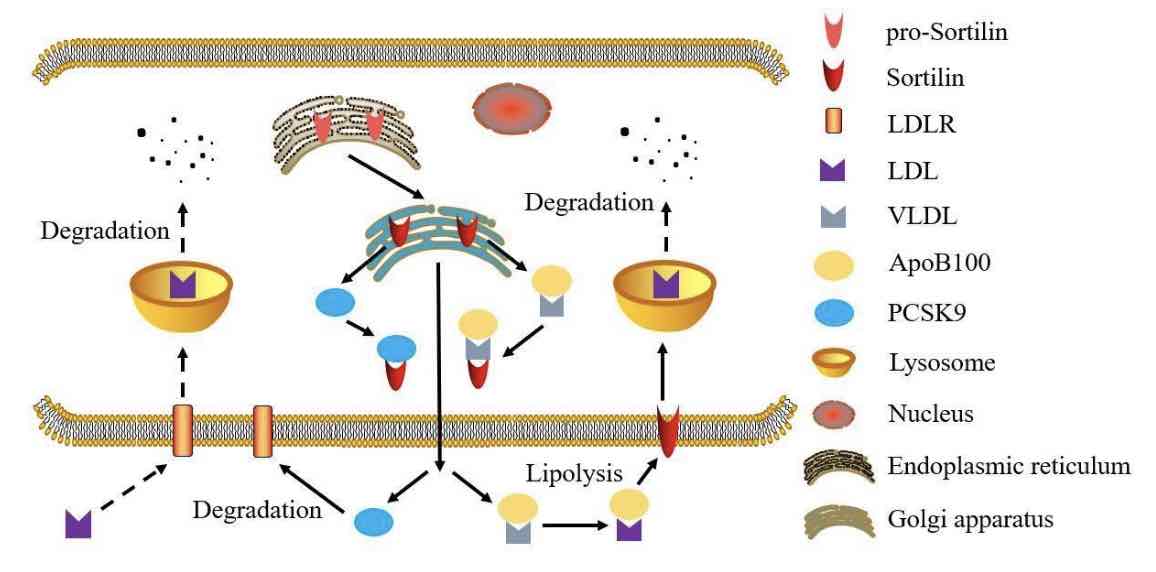
Downloads
Additional Files
Published
License
Copyright (c) 2021 Xin Su, Xiang Chen, Bin Wang, Linjian Chen, Cuilian Dai

This work is licensed under a Creative Commons Attribution 4.0 International License.
How to Cite
Accepted 2021-10-28
Published 2022-06-01