Tenofovir-silver nanoparticles conjugate ameliorates neurocognitive disorders and protects ultrastructural and cytoarchitectonic properties of the prefrontal cortex in diabetic rats
DOI:
https://doi.org/10.17305/bjbms.2021.6699Keywords:
Tenofovir, Prefrontal cortex, silver nanoparticles, neuroinflammation, neurological dysfunctionAbstract
Tenofovir disoproxil fumarate (TDF) is the highly recommended antiretroviral drug in human immunodeficiency virus management. Although research has shown the neurological and metabolic disorders associated with TDF administration, the effect of TDF-silver nanoparticles conjugate (TDF-AgNPs) on the disorders has not been fully elucidated. Thus, this study evaluated the neuroprotective effects of TDF-AgNPs on ultrastructural and cytoarchitectonic properties of the prefrontal cortex (PFC) in diabetic rats. Forty-two adult male Sprague-Dawley rats (250 ± 13 g) were randomly divided into non-diabetic groups (1-3) and diabetic groups (4-6), each administered distilled water (0.5 ml/100g, p.o), TDF (26.8 mg/kg/bw, p.o) or TDF-AgNPs (6.7 mg/kg, i.p). After eight weeks of administration, cognitive function, oxidative injury and tissue inflammation were evaluated. Also, PFC ultrastructure was observed using transmission electron microscopy, Nissl staining and immunohistochemistry. Diabetic rats administered TDF exhibited cognitive deficits; and increases in blood glucose, malondialdehyde and interleukin-1 beta (IL-1β) levels, which correlate with decreases in glutathione level, and superoxide dismutase (SOD) and catalase activities. Furthermore, loss of PFC astrocytes and neuronal organelles was observed. Conversely, TDF-AgNPs administration to diabetic rats improved cognitive deficits; and increased glutathione, SOD, and catalase, but reduced PFC malondialdehyde and IL-1β concentrations. Notably, TDF-AgNPs prevented loss of PFC neurons and astrocytic cells, and morphology aberration of neuronal organelles. This study suggests that TDF-AgNPs attenuated cognitive deficits via silver nanoparticles' antioxidant and anti-inflammatory properties, preventing the loss of PFC astrocytes and neurons. The TDF-AgNPs may be utilized to ameliorate the neurological dysfunction caused by prolonged TDF administration.
Citations
Downloads
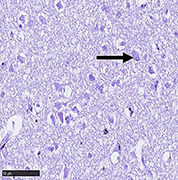
Downloads
Additional Files
Published
Issue
Section
Categories
License
Copyright (c) 2022 SODIQ LAWAL, Samuel Oluwaseun Olojede, Sheu Oluwadare Sulaiman, Okikioluwa Stephen Aladeyelu, Roshila Moodley, Edwin C. Stephen Naidu , Carmen Olivia Rennie, Onyemaechi Okpara Azu

This work is licensed under a Creative Commons Attribution 4.0 International License.
How to Cite
Accepted 2021-12-05
Published 2022-07-29