Strategies and safety considerations of booster vaccination in COVID-19
DOI:
https://doi.org/10.17305/bjbms.2021.7082Keywords:
SARS-CoV-2, COVID-19 vaccine, Omicron, booster vaccination, heterologous vaccinationAbstract
The first-generation SARS-CoV-2 vaccines have played a significant role in controlling the COVID-19 pandemic, preventing severe diseases, and reducing mortality. However, the continuous emergence of SARS-CoV-2 variants, the persistence of breakthrough infections, and the seemingly rapid decline in the protective efficacy of SARS-CoV-2 vaccines have presented additional challenges for the next phase. There is an urgent need to confirm the necessity of further booster vaccination and combination vaccine approaches. This paper summarizes the latest literature on SARS-CoV-2 variants and vaccine effectiveness and concludes that it is essential to implement booster immunization strategies. Priority should be given to high-risk groups, the elderly, and immunocompromised people. In addition, heterologous vaccination has a longer duration of effect and a broader spectrum than homologous vaccination, making it more conducive to managing the immune escape of SARS-CoV-2 variants.
Citations
Downloads
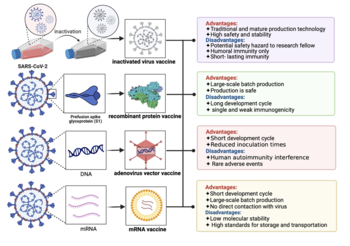
Downloads
Additional Files
Published
Issue
Section
Categories
License
Copyright (c) 2022 Hanyan Meng, Jianhua Mao, Qing Ye

This work is licensed under a Creative Commons Attribution 4.0 International License.
How to Cite
Accepted 2022-03-27
Published 2022-06-01